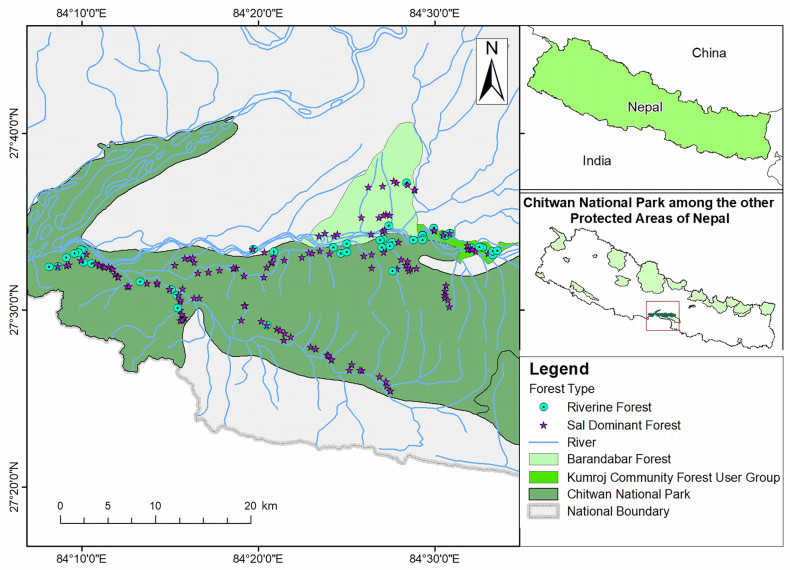
Citation: | Hani Amir Aouissi, Mostefa Ababsa, Aissam Gaagai, Zihad Bouslama, Yassine Farhi, Haroun Chenchouni. 2021: Does melanin-based plumage coloration reflect health status of free-living birds in urban environments?. Avian Research, 12(1): 45. DOI: 10.1186/s40657-021-00280-7 |
Ecological functions and processes in urban ecosystems are governed by various human activities. City-adapted and city-exploiting animal species are expected to present certain specific behavioral and physiological traits in comparison to city-avoiders or conspecific individual frequenting less urbanized or rural environments. A trait of high importance, the plumage color polymorphism has been selected as the main study model and was correlated with different morphological and physiological parameters to highlight its importance in determining the possible health status of urban Feral Pigeons (Columba livia) in North African urban habitats.
Different body morphometrics, hematological and hemoparasitic parameters were quantified on free-living Feral Pigeons in urban environments of northern Algeria. Moreover, plumage melanin-based coloration (MBC) was measured and the data collected at the individual scale was correlated with the previous parameters using linear and non-linear modeling approaches.
Plumage MBC scores of the sampled Feral Pigeons ranged between 0.3% and 74.8%. Among the 12 morphological traits measured, body weight, tail length and total length were deemed to be positively correlated with MBC. Darker morphs appeared to have more hemoparasites compared to lighter pigeons. Quite the same observation goes with the immunity but with non-linear trends. The number of monocytes and granulocytes increased with the increase in MBC levels in lighter morphs, while pigeons with high MBC scores exhibited negative relationships between MBC levels and the number of white blood cells.
Despite the existence of a number of studies demonstrating phenotypic directional selection, further studies are undoubtedly necessary to understand in detail the underlying mechanisms in species life-history strategies between differently colored individuals. Findings of this correlative study open exciting perspectives revealing that MBC can be considered a good indicator of and health status and adaptation strategies to changes in urban environments.
Of all vertebrate animals, birds are most likely to group and move together with other species (Morse et al. 1977; Goodale et al. 2017). Mixed-species flocks of birds (MSFs) reach their peak of diversity in forested habitat, where they are found, year-round in the tropics, and in migration and winter in temperate regions (Greenberg 2000; Goodale et al. 2017). Forest MSFs have been described on all continents except Antarctica, with at least 170 descriptive studies over a century of research (Zou et al. 2018). Participation in MSFs helps dilute predation risk (Beauchamp 2014), and increase foraging efficiency without increasing competition as much as monospecific flocks (Sridhar et al. 2009; Goodale et al. 2017). However, mixed-species flocking may also have costs, including that species may have to move at speeds or in locations that are not optimal for them (Hutto 1988; Darrah and Smith 2013).
A frequent observation about MSFs is that some 'nuclear' species are more important than others to their formation or maintenance, and tend to lead them (Moynihan 1962; Zou et al. 2018). These nuclear species are keystone species for their communities: essential to their functioning and conservation (Mills et al. 1993). Nuclear species tend to be gregarious and to engage in conspicuous calling and otherwise active behavior (Hutto 1994; Goodale and Beauchamp 2010; Pagani-Nunez et al. 2018). Because they have an important conspecific audience in MSFs, which may include kin or mates, it is thought these species are likely to produce costly information such as alarm calls (Goodale et al. 2020).
However, whether a species is a nuclear species is not necessarily black-and-white. In some systems, for example, there are 'secondary' leaders that appear to gain importance when the primary nuclear species is absent (Diamond 1987; Weeks et al. 2020), whereas in other systems there may be several redundant nuclear species (Jones and Robinson 2021). Another complex issue involves a 'sentinel species', one that is particularly vigilant about predation risk, such as drongos (Family Dicruridae) in Asia (Goodale et al. 2020), and whether such a species should be considered nuclear or not. Although drongos make alarm calls to which other species respond, drongos can manipulate other species with false alarms (Flower et al. 2014), attract other species towards MSFs from which they are benefitted (Goodale and Kotagama 2006), and even kleptoparasitize other species (King and Rappole 2001a). It is not clear whether a bird that gives such a complex mix of benefits and costs should be considered a nuclear species, and thus it is necessary to search for more evidence of whether drongos are able to lead MSF systems.
There have been many MSF studies in the southern regions of the South Asia (specifically the Western Ghats and Sri Lanka; Goodale et al. 2009), and some systems have been described in Northeastern India (Srinivasan et al. 2012) and central Myanmar (King and Rappole 2001b). However, to our knowledge there have been no formal descriptions of MSFs in Nepal. This is despite an exceptionally rich avifauna of 887 species, a large ornithological literature, and a strong bird conservation ethos and community (Inskipp et al. 2016; Baral et al. 2018). As an introductory study, we initially aimed to describe MSFs inside and around Chitwan National Park, Nepal's oldest national park and an Important Bird Area for the country (Inksipp et al. 2016), surveying the two most important forest types of the areas (CNP 2015). Preliminary observations, however, suggested that usual gregarious nuclear species were not common, and so we refined our goals to include investigating the leadership of MSFs and especially the role of drongos.
Chitwan National Park (CNP; 27°16′–27°42′ N, 83°50′–84°46′ E, elevation 150–815 m a.s.l., Fig.1) was established in 1973, and has been documented to provide habitat for 643 species of birds. The 953 km2 park has an additional buffer zone of 729 km2 and is adjacent to other forested areas such as Barandabhar Forest and Kumroj Community Forest (NTNC-BCC and CNP 2020). This larger area, in which we worked, includes the Narayani, Rapti and Reu rivers and their many tributaries. The climate of the region is tropical and subtropical, with a strong summer monsoon; average temperature ranges from 8 ℃ to 35 ℃, and mean annual rainfall is ~ 2600 mm (CNP 2015).
We concentrated on sampling two habitats: Sal Forest (SF) and riverine forest (RF). SF is the most common forest type of the park, covering ~ 70% of its area. It is dominated by Sal (Shorea robusta), intermixed with Asna (Terminalia alata), Barro (T. bellerica), Bhalayo (Semecarpus anacardium), Karam (Adina cardifolia), Kutmero (Litsea monipetala), and Tantari (Dillenia pentagyna) (Thapa 2011; Baral et al. 2020), with sparse understory ground cover (Lehmkuhl 1994). In contrast, RF is not very common (~ 7% of the area), but is structurally different, having higher density of trees per hectare, basal area, and tree species richness than SF (Engstrom et al. 2020), and floristically distinct, dominated by Vellar (Trevia nudiflora), Khayar (Acacia catechu), Sissoo (Dalbergia sisso) and Simal (Bombax ceiba). Other habitats of the park are composed of grasslands or wetlands and would not be expected to have forest bird flocks, as grassland and wetland flock systems are very distinct from those of forest (Goodale et al. 2017).
As part of a larger study on the flocking species the Greater Necklaced Laughingthrush (Garrulax pectoralis) and the Lesser Necklaced Laughingthrush (Garrulax monileger), we aimed to characterize the composition and organization of MSFs of the region and the two habitats. Field studies were conducted from October 2019 to January 2020. We walked dirt roads and footpaths in the park and its surroundings, between 07:30–12:00 h and 14:30–17:30 h and avoided revisiting the same roads, with the objective of sampling as many parts of the study area as possible. An MSF was defined as a group of birds moving in the same direction for more than five minutes (Goodale et al. 2009), and was observed from one point for 13.0 ± 8.2 (mean ± standard deviation [SD]) min on average, and for a maximum of 30 min. All species seen as moving with the MSF at any one moment in time were included in its composition. When a species was seen foraging, its vertical strata was also recorded visually, as terrestrial, understory (bottom third of forest height), midstory (middle third) or canopy (topmost third). We also noted the order that species crossed gaps (trails, open water, or forest gaps), making only one observation per MSF. All observations were made by one observer (KRG). Taxonomy follows Grimmett et al. (2016).
For the analysis, we classified species by their traits, using data from the published literature, as well as from the field. Birds were categorized as to their diet (carnivores, frugivores [including granivores], insectivores, nectarivores, omnivores), using data from Grimmett et al. (2016) and the Birds of the World (BOW; https://birdsoftheworld.org/bow/home). Insectivores and omnivores that consume a lot of insects were further classified into different foraging techniques (gleaning, probing, sallying; Remsen and Robinson 1990). Bird size was categorized as small (less than 10 cm head to tail), medium (11–20 cm) and large (above 20 cm), and used the same data sources as diet. We categorized species as to their abundance (regular, common, uncommon and rare; categories follow Machado [ 1999]), and migration ecology (resident and migrant, including altitudinal migrants), based on regional studies of the park's avifauna (NTNC-BCC and CNP 2020). Vertical stratification (understory, midstory, canopy, and 'all levels' [there was no clear majority in one vertical strata]) came from field data. As data were heteroschedastic, we used Welch's T-tests to compare the characteristics of MSFs between the two habitats. Mean values are shown ± SD.
To identify MSF types, we used hierarchical cluster analysis with Ward's minimum variance method on a matrix of species presence and absence (e.g., King and Rappole 2001b; Srinivasan et al. 2012). In order to characterize MSFs, we calculated the mean body size of all the species in an MSF, and then categorized MSFs into those with large, medium and small mean body sizes using the same thresholds of size as above. We also categorized the MSFs into the dominant vertical strata of the species in them. We then used Fisher Exact Tests to determine whether different characteristics were spread non-randomly among clusters. We also asked through Fisher Tests whether certain families or species were over-represented in one cluster more than the others. P-values < 0.05 were considered significant.
During the study period, we encountered a total of 222 MSFs (162 in SF and 60 in RF), including 100 species (93 species in SF, 75 in RF) and 6097 individuals (4509 in SF, 1588 in RF). The mean species richness of MSFs was 9.5 ± 3.6, and the mean number of individuals was 27.6 ± 15.8. There was no difference between the SF and RF habitats in MSF species richness, the number of individuals, or MSF Shannon–Wiener diversity (t-values < 0.72, P-values > 0.48).
Eighteen species were regular species in MSFs (seen in > 25% of MSFs in at least one habitat; Table 1), and of these all but one (Greenish Warbler Phylloscopus trochiloides) was a resident; 16 were insectivores. Likewise, of all the species observed in MSFs (see Additional file 1: Table S1 for a full list), 88% were residents, and 81% were insectivores, with the next most common category being frugivores at 11%. Among the insectivores, gleaners (63%) represented the majority, with salliers next most frequent at 23.5%. When considering vertical stratification, species that inhabited the canopy were the most common (42%), with understory (26%) and midstory (23%) species about equally represented.
Species | Occurrence | FG | FM | VS | MB | Mean No. of individuals±SD | ||
SF | RF | |||||||
Scarlet Minivet (Pericrocotus speciosus) | 87 | 22 | I | G | Can | R | 5.5±5.4 | |
Black Hooded Oriole (Oriolus xanthornus) | 73 | 28 | F | G | Can | R | 1.1±0.6 | |
Greater Racket Tailed Drongo (Dicrurus paradiseus) | 64 | 11 | I | S | Ms | R | 1.3±0.6 | |
Chestnut Bellied Nuthatch (Sitta cinnamoventris) | 51 | 27 | I | G | Can | R | 2.3±1.5 | |
Bronzed Drongo (Dicrurus macrocercus) | 51 | 6 | I | S | Can | R | 1.9±0.7 | |
Grey Capped Pygmy Woodpecker (Dendrocopos canicapillus) | 49 | 23 | I | P | Can | R | 1.8±0.7 | |
Jungle Babbler (Turdoides striata) | 47 | 10 | I | G | Ms | R | 9.9±4.3 | |
Great Tit (Parus major) | 45 | 15 | I | G | Ms | R | 1.7±1.1 | |
Greenish Warbler (Phylloscopus trochiloides) | 43 | 18 | I | G | All | M | 6.7±4.4 | |
Grey Headed Woodpecker (Picus canus) | 43 | 6 | I | P | All | R | 1.3±0.5 | |
Large Cuckooshrike (Coracina macei) | 43 | 13 | I | S | Can | R | 1.1±0.3 | |
Velvet Fronted Nuthatch (Sitta frontalis) | 43 | 12 | I | G | Can | R | 1.9±1.2 | |
Bar Winged Flycatcher Shrike (Hemipus picatus) | 42 | 7 | I | S | Can | R | 5.3±6.2 | |
Fulvous Breasted Woodpecker (Dendrocopos macei) | 41 | 21 | I | P | Can | R | 1.5±0.8 | |
Rufous Treepie (Dendrocitta vagabunda) | 41 | 13 | C | G | Ms | R | 2.0±0.6 | |
Common Iora (Aegithina tiphia) | 36 | 16 | I | G | Can | R | 3.4±3.8 | |
Hume's Leaf Warbler (Phylloscopus humei) | 36 | 16 | I | G | All | R | 1.2±0.7 | |
Greater Goldenback (Dinopium benghalense) | 33 | 17 | I | P | All | R | 1.8±1.1 | |
Species are ordered by the overall number of MSFs in which they were found. FG feeding guild, FM foraging method, VS vertical strata, MB migratory behavior, F frugivore, I insectivore, O omnivore, G gleaner, P prober, S sallier, All all levels, Can canopy, Ms midstory |
We also encountered 35 species that never participated in MSFs in the two forest types, 32 in SF and 21 in RF (Additional file 1: Table S2). Here 46% were frugivores and insectivores was the next most common group at 37%. Non-flocking species were significantly less likely to be insectivores than flocking ones (Fisher's Exact Test, P < 0.0001).
Hierarchical clustering divided the 222 MSFs into three main clusters (Fig.2). These clusters were distinguishable in their characteristics with Cluster 1 having MSFs with large mean body sizes more often (58/74, 78%) than the two other clusters (84/148, 57%; Fisher's Exact Test, P = 0.0018). However, the clusters did not differ as to their inclusion of species of different diets, vertical strata or habitats (SF vs. RF). Composition also differed among clusters. Cluster 1 had more Dicruridae than in other two clusters (48/74, 65% vs. 74/148, 50%; Fisher's Exact Test, P = 0.04), and specifically the Greater Racket-tailed Drongo (39/74, 53%, vs. 34/148, 23%; Fisher's Exact Test, P < 0.0001). Cluster 2 had more Phylloscopidae than the other two clusters (51/89, 57% vs. 39/133, 29%; Fisher's Exact Test, P < 0.0001).
We recorded a movement across a gap for 61 of the 222 MSF observations, only taking one observation per MSF. Greater Racket-tailed Drongo led the most MSFs in both habitats (Table 2). Scarlet Minivet (Pericrocotus flammeus), Bronzed Drongo (Dicrurus aeneus), Spangle Drongo (Dicurus hottentotus) and Bar-winged Flycatcher Shrike (Hemipus picatus) also led MSFs. Some species not only did not lead many MSFs but were consistently seen towards the end of the MSFs, especially six species of woodpeckers.
Sal forest | Riverine forest | ||||||
Leader species | % occasions led (n=39) | Leader species | % occasions led (n=22) | ||||
Greater Racket-Tailed Drongo | 35.9 | Greater Racket-Tailed Drongo | 18.2 | ||||
Scarlet Minivet | 10.3 | Spangled Drongo (Dicrurus bracteatus) | 18.2 | ||||
Bar-Winged Flycatcher Shrike | 7.7 | Scarlet Minivet | 13.6 | ||||
Bronzed Drongo | 7.7 | Oriental White Eye (Zosterops palpebrosus) | 13.6 | ||||
11 other species | ≤2 leading events | 7 other species | ≤2 leading events | ||||
Only species that led MSFs at least three times in one of the habitats are listed. For scientific names, see Table 1 |
The Chitwan MSFs that we described are similar to terrestrial forest MSFs globally in that the participants are mostly insectivorous (Powell 1985; Goodale et al. 2017), and not terrestrial (Thiollay 1999). They are also dominated by leaf-gleaning species. Their movement through the vegetation disturbs insects that a group of sallying species like drongos take advantage of (Satischandra et al. 2007; Sridhar and Shanker 2014).
The Chitwan MSFs also have some patterns that appear widespread in Asia. There were a significant number of gregarious species, so that there were more than three times the number of individuals in an average MSF than the number of species. A high number of individuals per MSF has been seen as a characteristic of MSFs of South Asia (Goodale et al. 2009), East Asia (Goodale et al. 2015), and Melanesia (Diamond 1987; Weeks et al. 2017, 2020), due to the presence of highly gregarious nuclear species or secondary leaders. In contrast, MSFs of the Neotropics often include only one or two individuals of most species (Powell 1985). The low number of migrants in MSFs seems to be fairly widespread in South Asia (Goodale et al. 2009); in contrast, some areas of the neotropics have MSFs that are dominated by migrants (e.g., Hutto 1987). The overall picture here is thus that MSFs in different parts of the world with distinct evolutionary histories can show quite different features.
Composition wise, these MSFs are dominated by birds of the canopy (e.g., minivets (Genus Pericrocotus, Family Campephagidae), and ioras (Family Aegithinidae). This is reminiscent of certain MSF types that have been described in Northeast India (the 'canopy' cluster of Srinivasan et al. 2012) and Myanmar (the 'cuckooshrike' cluster of King and Rappole 2001b). Similar MSFs with minivets have been described as far away as peninsular Malaysia (McClure 1967). The clustering result suggests that there may be separate MSF types in the region that are segregated by size, a result also found in other studies in the region (King and Rappole 2001b; Srinivasan et al. 2012). Both meta-analyses (Sridhar et al. 2012) and field studies (Mammides et al. 2018) have shown the birds tend to associate in MSFs with other species of the same body size.
One of our questions entering this study was whether there would be a difference between Sal and Riverine forests habitat types, since the vegetation is structurally and floristically distinct (Lehmkuhl 1994; Thapa 2011; Engstrom et al. 2020). However, we found that MSFs in the two habitats were highly similar, with no significant differences in MSF size and composition. Likewise, Engstrom et al. (2020) found no significant differences in bird species richness between the two habitats. Engstrom et al. (2020) did find that some common species like Pale-chinned Flycatcher (Cyornis poliogenys), Black Bulbul (Hypsipetes leucocephalus) and Blue-throated Barbet (Psilopogon asiaticus) were seen only in RF, but these species are not common in MSFs.
Connected to the dominance of canopy species, these MSFs did not have a dominant gregarious nuclear species in the understory or midstory. In Asia, nuclear species are often of the babbler family, and the ability of their many individuals to forage at a range of heights enables many other species to follow them (Hsieh and Chen 2011). For example, Turdoides genus babblers and Alcippe genus fulvettas lead MSFs in South Asia (Kotagama and Goodale 2004) and East Asia (Zhou et al. 2019), respectively, but here species of these genera were fairly rare (< 30% of MSFs).
We hypothesize that this lack of gregarious nuclear species provides an opportunity for drongos to lead MSFs. We repeatedly observed drongos perched for a minute or so, sally and catch food, return to the perch, and then fly ahead of the MSF, with the flock eventually following. Drongos were suggested to be nuclear species in an earlier observation in Sri Lanka in an area which the regular Turdoides nuclear species was absent (Perera et al. 2016). However, the observations here are the first we know to show drongos can be the primary leaders for an MSF system. Yet it has already been established that drongos are important to other species in MSFs. Their alarm calls are especially reliable (Goodale and Kotagama 2005a) and are responded to by many species (Goodale and Kotagama 2008). Further, playback of drongos is as attractive as playback of gregarious babblers to other flock participants in Sri Lanka (Goodale and Kotagama 2005b), and drongos also are known to manipulate other species by attracting them with vocal mimicry (Goodale and Kotagama 2006). So, it makes sense that in a system without dominant gregarious species, drongos can turn into the most important nuclear species.
In these observations in Nepal, we also did not see any kleptoparasitism by drongos, but it was clear that they made alarm calls. Hence, perhaps the benefits they give to other species (vigilance) overweigh the costs (kleptoparasitism, behavioral manipulation). We found that one cluster of MSFs was distinguished by the presence of Greater Racket-tailed Drongos, and this cluster was also characterized by larger body size species. These observations suggest that the presence of drongos is important to the composition of flocks and to community assembly in this flock system. The phylogenetic work of Péron (2017) has also suggested that drongos are important to the assembly to MSFs in the Old World: MSFs with drongos were more phylogenetically clustered than those without, perhaps because the drongos give vigilance benefits that compensates for competition between more closely related species.
These observations are significant because it demonstrates that sentinel species—species that are particularly vigilant—can lead MSFs on different continents. The antshrikes (Thamnomanes sp.) have been shown to be especially important to the formation and leadership of Amazonian MSFs (Stotz 1993; Martínez et al. 2018). Like drongos, these species sally for their prey, which makes them particularly vigilant (Munn 1984), and other species listen to their alarm calls (Martínez et al. 2016), although they are capable of making false alarm calls to steal food as do drongos (Munn 1986). Thus drongoand antshrikeled MSFs seem to be examples of the convergent evolution of similar community organization in bird MSFs with different evolutionary histories.
In recent years, there has been a greater emphasis on community-wide conservation, trying to conserve the interactions between species. Mixed-species flocks can be part of such a strategy; indeed, nuclear species can be targeted specifically to try to conserve other participants in MSFs (Zou et al. 2018). Nepal has been successful in focusing on its endangered species in conservation studies (Baral et al. 2018). We hope that this small study can be a starting point for more research on the sociality of Nepali birds, and how this factor can be incorporated into the country's strategy for bird conservation.
The online version contains supplementary material available at https://doi.org/10.1186/s40657-021-00292-3.
Additional file 1: Table S1. A list of all species that participated in MSFs during the survey and their traits. Table S2: A list of all species seen during the survey but not in MSFs.
We thank Tikaram Giri for providing insight on the birds of Chitwan National Park and providing warm hospitality in his hotel. We thank Bishnu Mahato, Pushan Mahato, Anish Mahato and Yam Mahato for their assistance in the field, Asst. Prof. Ramesh Sapkota for his support in statistical analysis and Mrs. Neeru Maharjan Gosai for providing moral support to the first author during the entire research period. We also thank Tej Kumar Shrestha, Lumbini Environmental Services Pvt. Ltd., Nepal for providing a working space during the write-up of the manuscript. Three anonymous reviewers provided helpful comments that improved the manuscript.
KRG and EG conceived and designed the study. KRG collected the data and conducted the analysis. KRG and EG wrote the article. Both authors read and approved the final manuscript.
The primary datasets are included in the manuscript and supplemental information.
This study was purely observational, without effects on the birds involved. We obtained permission to conduct the research from the Department of National Park and Wildlife Conservation of Nepal, Chitwan National Park, and the authorities of the community forests.
Not applicable.
The authors declare they have no conflicts of interest.
Aouissi HA, Belabed AI, Bouslama Z. Doves' mapping and inventory into the urban sites of Annaba (Northeastern of Algeria). Adv Environ Biol. 2015;12: 328–38.
|
Aouissi HA. Écologie des espèces aviaires dans le tissu urbain de la ville de Annaba. Doctoral Thesis. Annaba: University of Annaba; 2016.
|
Berry JL. Urbanization. The earth as transformed by human action. In: Turner II BL, Clark WC, Kates RW, Richards JF, Mathews JT, Meyer WB, editors. The earth as transformed by human action: global and regional changes in the biosphere over the past 300 years. Cambridge: Cambridge University Press, UK; 1990. p. 103–19.
|
Borras A, Pascual J, Senar JC. What do different bill measures measure and what is the best method to use in granivorous birds? J Field Ornithol. 2000;71: 606–11.
|
Brazil M. Birds of east Asia: China, Taiwan, Korea, Japan, and Russia. Princeton, New Jersey: Princeton University Press; 2009.
|
Campbell TW. Avian hematology and cytology. 2nd ed. Ames, IA: Iowa State University Press; 1995.
|
Chanarin I. Hematology: principles and procedures. J Clin Pathol. 1984;37: 1419.
|
Chedad A, Bendjoudi D, Beladis I, Guezoul O, Chenchouni H. A comprehensive monograph on the ecology and distribution of the House bunting (Emberiza sahari) in Algeria. Front Biogeogr. 2021;13: e47727.
|
Chenchouni H. Contribution à l'étude de la bio-écologie de la Cigogne blanche (Ciconia ciconia) dans la région de Batna (Nord-est algérien). Doctoral Thesis. Algeria: University of Batna; 2017a.
|
Combes C. Parasitism: the ecology and evolution of intimate interactions. Chicago: University of Chicago Press; 2001.
|
Davidsohn I, Henry JB. Clinical diagnosis by laboratory methods. 15th ed. Philadelphia, Pa: W. B. Saunders Co; 1974.
|
de Godoi FSL, Nishi SM, de Jesus Pena HF, Gennari SM. Toxoplasma gondii: diagnosis of experimental and natural infection in pigeons (Columba livia) by serological, biological and molecular techniques. Rev Bras Parasitol Vet. 2010;19: 238–43.
|
Eck S, Fiebig J, Fiedler W, Heynen I, Nicolai B, Töpfer T, et al. Measuring birds/vögel vermessen. Germany: Deutsche Ornithologen-Gesellschaft; 2011.
|
Emaresi G, Henry I, Gonzalez E, Roulin A, Bize P. Sexand melanism-specific variations in the oxidative status of adult tawny owls in response to manipulated reproductive effort. J Exp Biol. 2016;219: 73–9.
|
George EL, Panos A. Does a high WBC count signal infection? Nursing. 2005;35: 20–1.
|
Hart BL. Behavioural defence. In: Clayton DH, Moore J, editors. Host-parasite evolution: general principle and avian models. Oxford: Oxford University Press; 1997. p. 59–77.
|
Hawkey CM, Dennett TB. A colour atlas of comparative veterinary haematology. London: Wolfe Publishing; 1989.
|
Hill GE, McGraw KJ. Bird colouration: function and evolution. Cambridge: Harvard University Press; 2006.
|
Jacquin L. Coloration mélanique et stratégies d'histoire de vie chez le pigeon biset urbain. Doctoral Thesis. University of Paris 6; 2011.
|
Jarry G, Baillon F. Hivernage de la tourterelle des bois (Streptopelia turtur) au Sénégal: étude d'une population dans la région de Nianing. Paris: Report of CRBPO; 1991.
|
Johnston RF, Janiga M. Feral pigeons. Vol. 4. Oxford: Oxford University Press; 1995.
|
Kark S, Iwaniuk A, Schalimtzek A, Banker E. Living in the city: can anyone become an 'urban exploiter'? J Biogeogr. 2007;34: 638–51.
|
Luniak M. Synurbization – adaptation of animal wildlife to urban development. In: Shaw WW, Harris K, Vandruff L, editors. Proceedings of the 4th international symposium on urban wildlife conservation. Tucson, Arizona: University of Arizona; 2004. p. 50–5.
|
Madans JH, Webster KM. Health surveys. In: Wright JD, editor. International encyclopedia of the social and behavioral sciences. 2nd ed. Orlando: University of Central Florida; 2015. p. 725–30.
|
Marzluff JM. Worldwide urbanization and its effects on birds. In: Marzluff JM, Bowman R, Donnelly R, editors. Avian ecology and conservation in an urbanizing world. Boston: Springer; 2001. p. 19–47.
|
Périquet JC. Le Pigeon: races, élevage et utilisation, reproduction, hygiène et santé. Collection Les cahiers de l'élevage, Ed. Rustica, Paris; 1998.
|
Rasmussen PC, Anderton JC. Birds of south Asia: the Ripley guide: attributes and status. 1st Edn, Vol. 2. Washington and Barcelona: Smithsonian Institution and Lynx Edicions; 2005.
|
Stöppler MC, Shiel WC, Credo Reference (Firm), WebMD (Firm). Webster's new world medical dictionary, 3rd ed.; Redo Reference: Boston, MA, USA; Wiley: Hoboken, NJ, USA. 2014; 480 p.
|
1. | Umamageswari T.S.R. GIS based ground water assessment of Nilakkottai Taluk, Tamil Nadu, India: hydrogeochemistry and statistical perspective. Journal of Environmental Engineering and Landscape Management, 2025, 33(1): 30. DOI:10.3846/jeelm.2025.22950 |
2. | Javiera Arcila, Isaac Peña-Villalobos, Catalina B. Muñoz-Pacheco, et al. Urbanization's hidden influence: Linking landscape alterations and feather coloration with pigeon's cholesterol levels. Environmental Research, 2025, 271: 121115. DOI:10.1016/j.envres.2025.121115 |
3. | Ammari Abdessattar, Fartas Fadhila, Samir Kateb. Quality assessment using water quality indicators with geospatial analysis of groundwater quality El-Oued region, Northern Sahara, Algeria. Water Supply, 2024, 24(5): 1689. DOI:10.2166/ws.2024.090 |
4. | Rachid Chaibi, Nora Mimoune, Farouk Benaceur, et al. Extrinsic and intrinsic drivers of prevalence and abundance of hard-bodied ticks (Acari: Ixodidae) in one-humped camel (Camelus dromedarius). Parasite Epidemiology and Control, 2024, 27: e00387. DOI:10.1016/j.parepi.2024.e00387 |
5. | Aissam Gaagai, Hani Aouissi, Selma Bencedira, et al. Application of Water Quality Indices, Machine Learning Approaches, and GIS to Identify Groundwater Quality for Irrigation Purposes: A Case Study of Sahara Aquifer, Doucen Plain, Algeria. Water, 2023, 15(2): 289. DOI:10.3390/w15020289 |
6. | Nuela Manka'a Che-Ajuyo, Boye Liu, Zhuqing Deng, et al. Sex-biased, but not plumage color-based, prevalence of haemosporidian parasites in free-range chickens. Parasitology International, 2023, 93: 102722. DOI:10.1016/j.parint.2022.102722 |
7. | Aswathy Ashokan, Ratheesh Kumar R, Vidya Shree Bharti. Anthropogenic litter on sandy beaches in Mumbai Coast, India: a baseline assessment for better management. Arabian Journal of Geosciences, 2023, 16(1) DOI:10.1007/s12517-022-11155-6 |
8. | Ali Athamena, Aissam Gaagai, Hani Amir Aouissi, et al. Chemometrics of the Environment: Hydrochemical Characterization of Groundwater in Lioua Plain (North Africa) Using Time Series and Multivariate Statistical Analysis. Sustainability, 2022, 15(1): 20. DOI:10.3390/su15010020 |
9. | Walid Khelifi, Selma Bencedira, Marc Azab, et al. Conservation Environments’ Effect on the Compressive Strength Behaviour of Wood–Concrete Composites. Materials, 2022, 15(10): 3572. DOI:10.3390/ma15103572 |
10. | Aissam Gaagai, Hani Amir Aouissi, Andrey E. Krauklis, et al. Modeling and Risk Analysis of Dam-Break Flooding in a Semi-Arid Montane Watershed: A Case Study of the Yabous Dam, Northeastern Algeria. Water, 2022, 14(5): 767. DOI:10.3390/w14050767 |
11. | Jessica Diaz, Aspen T. Reese. Possibilities and limits for using the gut microbiome to improve captive animal health. Animal Microbiome, 2021, 3(1) DOI:10.1186/s42523-021-00155-8 |
Species | Occurrence | FG | FM | VS | MB | Mean No. of individuals±SD | ||
SF | RF | |||||||
Scarlet Minivet (Pericrocotus speciosus) | 87 | 22 | I | G | Can | R | 5.5±5.4 | |
Black Hooded Oriole (Oriolus xanthornus) | 73 | 28 | F | G | Can | R | 1.1±0.6 | |
Greater Racket Tailed Drongo (Dicrurus paradiseus) | 64 | 11 | I | S | Ms | R | 1.3±0.6 | |
Chestnut Bellied Nuthatch (Sitta cinnamoventris) | 51 | 27 | I | G | Can | R | 2.3±1.5 | |
Bronzed Drongo (Dicrurus macrocercus) | 51 | 6 | I | S | Can | R | 1.9±0.7 | |
Grey Capped Pygmy Woodpecker (Dendrocopos canicapillus) | 49 | 23 | I | P | Can | R | 1.8±0.7 | |
Jungle Babbler (Turdoides striata) | 47 | 10 | I | G | Ms | R | 9.9±4.3 | |
Great Tit (Parus major) | 45 | 15 | I | G | Ms | R | 1.7±1.1 | |
Greenish Warbler (Phylloscopus trochiloides) | 43 | 18 | I | G | All | M | 6.7±4.4 | |
Grey Headed Woodpecker (Picus canus) | 43 | 6 | I | P | All | R | 1.3±0.5 | |
Large Cuckooshrike (Coracina macei) | 43 | 13 | I | S | Can | R | 1.1±0.3 | |
Velvet Fronted Nuthatch (Sitta frontalis) | 43 | 12 | I | G | Can | R | 1.9±1.2 | |
Bar Winged Flycatcher Shrike (Hemipus picatus) | 42 | 7 | I | S | Can | R | 5.3±6.2 | |
Fulvous Breasted Woodpecker (Dendrocopos macei) | 41 | 21 | I | P | Can | R | 1.5±0.8 | |
Rufous Treepie (Dendrocitta vagabunda) | 41 | 13 | C | G | Ms | R | 2.0±0.6 | |
Common Iora (Aegithina tiphia) | 36 | 16 | I | G | Can | R | 3.4±3.8 | |
Hume's Leaf Warbler (Phylloscopus humei) | 36 | 16 | I | G | All | R | 1.2±0.7 | |
Greater Goldenback (Dinopium benghalense) | 33 | 17 | I | P | All | R | 1.8±1.1 | |
Species are ordered by the overall number of MSFs in which they were found. FG feeding guild, FM foraging method, VS vertical strata, MB migratory behavior, F frugivore, I insectivore, O omnivore, G gleaner, P prober, S sallier, All all levels, Can canopy, Ms midstory |
Sal forest | Riverine forest | ||||||
Leader species | % occasions led (n=39) | Leader species | % occasions led (n=22) | ||||
Greater Racket-Tailed Drongo | 35.9 | Greater Racket-Tailed Drongo | 18.2 | ||||
Scarlet Minivet | 10.3 | Spangled Drongo (Dicrurus bracteatus) | 18.2 | ||||
Bar-Winged Flycatcher Shrike | 7.7 | Scarlet Minivet | 13.6 | ||||
Bronzed Drongo | 7.7 | Oriental White Eye (Zosterops palpebrosus) | 13.6 | ||||
11 other species | ≤2 leading events | 7 other species | ≤2 leading events | ||||
Only species that led MSFs at least three times in one of the habitats are listed. For scientific names, see Table 1 |