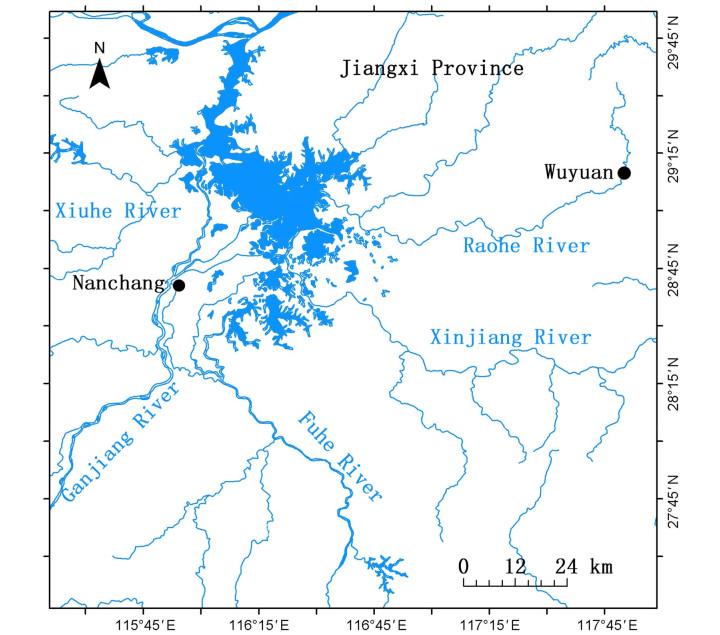
Citation: | Ailin Yang, De Chen, Pengcheng Wang, Yiqiang Fu, Zhengwang Zhang. 2017: Characterization of novel microsatellite markers of the Emei Shan Liocichla using restriction site-associated DNA sequencing. Avian Research, 8(1): 13. DOI: 10.1186/s40657-017-0071-8 |
The Emei Shan Liocichla (Liocichla omeiensis) is an endemic bird species to southwestern China with a small geographic range. However, little was known about the genetic status of this threatened species.
We applied restriction-site-associated DNA sequencing (RAD-Seq) for rapid mass identification of microsatellite markers of the Emei Shan Liocichla.
A total of 11, 564 microsatellite sequences were obtained, 600 random loci were designed for screening and 24 polymorphic microsatellite loci were selected for further validation. The average allele number, average observed heterozygosity and average expected heterozygosity were relatively low in our samples, which were 6.08, 0.6618 and 0.7048, respectively, indicating that the Emei Shan Liocichla might have lost some genetic diversity. Further analyses suggested that the populations distributed on two mountains (Daxiangling and Xiaoliangshan) showed a modest degree of genetic differentiation.
These novel microsatellite markers provided valuable preliminary knowledge regarding the genetic status of the Emei Shan Liocichla and can be useful in further studies, as well as in the management and conservation of this species.
For some waterfowl species, they use diving as the main foraging technique. Diving behavior of waterfowl may be affected by a number of different factors such as prey availability, its distribution, density and type (Benvenuti et al. 2002; Kato et al. 2003; Thompson and Price 2006), water temperature, quality and depth (Guillemette et al. 2004; Green et al. 2005; Thompson and Price 2006), as well as light levels (Wilson et al. 1993; Wanless et al. 1999), body size, physiological functions (Butler and Jones 1982; Sato et al. 2003; Halsey et al. 2006) and habitat type (Ropert-Coudert and Kato 2009). A number of studies have reported that across species, body mass is an important predictor of diving behavior (Trillmich et al. 1991; Costa 1993; Boyd and Croxall 1996; Schreer and Kovacs 1997; Watanuki and Burger 1999). Halsey et al. (2006) analyzed the diving ability of 195 species of birds and mammals and found that both dive and pause durations increased with body mass. Diving theory predicts that large-size birds with a potentially enormous capacity for oxygen storage and low metabolic rate, should be able to stay submerged and surface longer than smaller birds (Cooper 1986). Within a species, however, dive and pause duration of males and adults are usually longer than that of females and juveniles because of different body size (Duncan and Marquiss 1993; Kato et al. 1999; Polak and Ciach 2007; Gomes et al. 2009; Laich et al. 2012).
The Scaly-sided Merganser (Mergus squamatus) is listed in the first category of nationally protected wildlife species in China and is regarded as an endangered species by the IUCN in 2002 (Wang and Xie 2009). This species mainly breeds in southeastern Russia, North Korea and Heilongjiang, Jilin and Inner Mongolia in the northeast of China (Birdlife International 2014). Wintering areas include a wide stretch along the south bank of the Yangtze River (He et al. 2006), while the north of Jiangxi Province provides one of the major wintering habitats for this species in China. Its population is found mainly along four rivers of Jiangxi, i.e., the Xinjiang, Fuhe, Raohe and Xiuhe rivers and their tributaries (Liu et al. 2008; Zeng et al. 2013; Shao et al. 2014). At a global level, their number is about 1200 pairs of which about 250 individual birds are wintering in Jiangxi. At present, the population of the Scaly-sided Merganser continues to decline due to a very high level of habitat loss, illegal hunting and human interference (Birdlife International 2014 ). Although a number of previous studies about wintering ecology of the Scaly-sided Merganser have been carried out (Liu et al. 2008; Shao et al. 2010; Yi et al. 2010), relatively little is known about their diving behavior and the factors that impact this species during winter (Shao et al. 2014).
From December 2015 to March 2016, we studied the diving behavior of the wintering Scaly-sided Merganser in Wuyuan, Jiangxi. The objectives of this study were to (1) provide basic data on diving behavior of wintering Scaly-sided Merganser, and (2) reveal the effect of sex, temperature, time and flock size on its diving behavior.
Wuyuan County is located in the northeast of Jiangxi Province, China. This area has a humid subtropical climate with an average annual temperature of 16.7 ℃ (Shao et al. 2012) and the average annual precipitation is 1816 mm (Ji et al. 2014). Our study site was the Raohe River in Wuyuan County (29°9′24″-29°11′15″N, 117°50′01″‒117°51′06″E) (Zeng et al. 2013) (Fig. 1). The vegetation is dominated by broad-leaved deciduous forests and mixed broad-leaved and evergreen mountain forests. Scattered farmland and villages are distributed in this area. The main activity range of our merganser is focused on about 7 km of river banks. They are also found dispersed at other river sections or in company of other birds along other stretches of the river. The population of the Scaly-sided Merganser we studied consisted of about 20 individuals during one wintering period. Sympatric waterfowl species in this area include Chinese Spot-billed Duck (Anas poecilorhyncha), Mallard (Anas platyrhynchos), Mandarin Duck (Aix galericulata), Little Grebe (Tachybaptus ruficollis), Little Egret (Egretta garzetta), Green Sandpiper (Tringa ochropus), Common Sandpiper (Actitis hypoleucos) and Eurasian Coot (Fulica atra).
We used a focal sampling method to enumerate the diving activities of the Scaly-sided Merganser in the Wuyuan section of the Poyang Lake watershed, from December 2015 to March 2016. Data were collected with binoculars (30×) and a spotting scope (20‒60×) from 7:30 hours to 17:30 hours. In total 34 days of field investigations were carried out in our study area, involving 8 days in December, 7 in January, 9 in February and 10 days in March. In order to estimate the effect of various factors on the diving behavior of the merganser, we collected and compared diving data by sex, temperature (daily average air temperature), time (time of year and daytime) and flock size. We determined the sex by the feather color of its head or back. Data recorded include sex, temperature (once every half hour), flock size, start time and end time of diving, dive duration and pause time between consecutive dives. Diving efficiency, defined as the dive/pause (d/p) ratio, was calculated from the dive duration and the subsequent pause duration (Dewar 1924). We also tried to collect data at different time and from various observation points to decrease the possibility that we monitored the same individual bird more than once. In this paper, daytime means the different time period from 7:30 hours to 17:30 hours in diurnal scanning time.
One-way ANOVAs and LSD multiple comparisons were used to test the differences between each group (Jiang and Shao 2015). The Pearson correlation test was used to test the correlation between pause duration and the previous and subsequent dive duration. The Spearman correlation test was used to test correlations between temperature, time of year, daytime, flock size, dive and pause duration. All our calculated results are expressed as mean ± standard deviation (ˉx±SD), with p values < 0.05 considered significant. All statistical analyses were completed in Excel 2010 and SPSS 22.0.
A total of 201 focals and 37.14 h were performed and all focals were performed by the same person. During feeding, the Scaly-sided Merganser remained submerged for 2.5-34.7 s and paused on the surface between dives for 1.2-94.5 s, with diving efficiency ranging from 0.068 to 13.429. Mean dive duration was 18.8 ± 6.0 s (n = 4305) versus a mean pause duration of 12.9 ± 11.2 s (n = 4104), with a diving efficiency of 2.297 ± 1.626 (n = 4104) (Table 1). Pause duration was positively correlated with the previous dive duration (r = 0.099, p < 0.001, n = 4104), as well as with the duration of the subsequent dive (r = 0.094, p < 0.001, n = 4104).
Sex | Number of focals | Number of dives | Dive duration (s) | Number of pauses | Pause duration (s) | Diving efficiency |
Male | 103 | 2117 | 20.1 ± 6.2a | 2014 | 13.6 ± 12.0a | 2.401 ± 1.732a |
Female | 98 | 2188 | 17.5 ± 5.4b | 2090 | 12.2 ± 10.4b | 2.197 ± 1.510b |
Total | 201 | 4305 | 18.8 ± 6.0 | 4104 | 12.9 ± 11.2 | 2.297 ± 1.626 |
Values with the same letter indicate no significant difference (p > 0.05), while different letters indicate significant differences (p < 0.05), also applying to the tables below |
Mean dive duration (F = 223.373, df = 1, p < 0.001), mean time on the surface (F = 16.460, df = 1, p < 0.001) and diving efficiency (F = 16.189, df = 1, p < 0.001) of males were significantly longer or higher than the estimates for females (Table 1).
Significant differences were detected among the mean dive duration (F = 239.418, df = 2, p < 0.001) and mean time on the surface (F = 3.331, df = 2, p < 0.05) within the range of observed temperatures (Table 2). The mean dive duration was significantly longer when the temperature was 4-10 ℃ while was significantly shorter at 11-19 ℃. With the rise of temperature, the mean dive duration showed a significant trend of an initial decrease and a subsequent increase in submergence. Mean pause duration was highest when the temperature was 22-29 ℃ in contrast to the 4-10 ℃ range. With the rise of temperature, the mean pause duration showed no significant change. Diving efficiency was significantly higher when the temperature was 4-10 ℃ than at other temperatures, while it was lowest between 22 and 29 ℃. Dive duration was negatively correlated with the temperature (r = -0.269, p < 0.001) (Table 2).
Temperature (℃) | Number of dives | Dive duration (s) | Number of pauses | Pause duration (s) | Diving efficiency |
4‒10 | 1530 | 21.3 ± 5.5a | 1430 | 12.4 ± 10.1a | 2.579 ± 1.647a |
11‒19 | 1653 | 17.2 ± 5.5b | 1588 | 12.9 ± 11.4a | 2.176 ± 1.670b |
22‒29 | 1122 | 17.7 ± 6.1c | 1086 | 13.5 ± 12.3a | 2.105 ± 1.478b |
Significant differences were detected among the mean dive duration (F = 195.420, df = 3, p < 0.001) and the mean time on the surface (F = 23.652, df = 3, p < 0.001) over various months (Table 3). The mean dive duration in December and January was significantly higher than in February and March. The mean pause duration in January was significantly lower than in other months. Diving efficiency in January was significantly higher than in other months, contrast to that in February. The time of year from December 2015 to March 2016 was negatively correlated with dive duration (r = -0.176, p < 0.001) (Table 3).
Period/daytime | Number of dives | Dive duration (s) | Number of pauses | Pause duration (s) | Diving efficiency |
Month | |||||
Dec. | 842 | 21.1 ± 5.1a | 775 | 13.1 ± 8.2a | 2.136 ± 1.149a |
Jan. | 743 | 21.0 ± 6.3a | 705 | 9.7 ± 10.5b | 3.360 ± 1.985b |
Feb. | 1521 | 16.2 ± 5.3b | 1467 | 13.7 ± 11.2a | 1.903 ± 1.522c |
Mar. | 1199 | 19.1 ± 5.8c | 1157 | 13.7 ± 13.0a | 2.503 ± 1.983d |
Daytime | |||||
7:30‒8:59 | 421 | 19.4 ± 5.3a | 397 | 16.4 ± 13.1a | 1.976 ± 1.555a |
9:00‒15:59 | 3755 | 18.8 ± 6.0a | 3582 | 12.6 ± 11.0b | 2.350 ± 1.646b |
16:00‒17:30 | 129 | 14.9 ± 5.8b | 125 | 11.2 ± 9.8b | 1.811 ± 0.933a |
Significant differences were detected among mean dive duration (F = 29.517, df = 2, p < 0.001), mean time on the surface (F = 21.922, df = 2, p < 0.001) and diving efficiency (F = 21.922, df = 2, p < 0.001) at various daytime (Table 3). The mean dive duration and mean pause time between 7:30 and 8:59 was longest, while shortest between 16:00 and 17:30. Diving efficiency was highest between 9:00 and 15:59 hours, contrast to that between 16:00 and 17:30 hours. Daytime was negatively correlated with dive duration (r = -0.081, p < 0.001) and pause duration (r = -0.101, p < 0.001) while it was positively correlated with diving efficiency (r = 0.052, p = 0.001) (Table 3).
Significant differences were observed among mean dive duration (F = 106.694, df = 3, p < 0.001) and mean time on the surface (F = 5.106, df = 3, p < 0.05) for various flock sizes (Table 4). With flock sizes between 1 and 5 birds, the mean dive duration was significantly higher than that of any other flock size, while between 16 and 19 birds, the mean dive duration was significantly less than that of any other flock size. With an increase in the flock size, the mean dive duration showed a significantly decreasing trend. With flock sizes between 16 and 19 birds, the mean pause duration was significantly less than that of any other flock size. With an increase in flock size, the mean pause duration showed a gradually decreasing trend. Diving efficiency was significantly higher in flock sizes between 1 and 5 birds than in any other flock size, while between 11 and 15 birds, diving efficiency was significantly less than in any other flock size. Flock size was negatively correlated with dive duration (r = -0.259, p < 0.001) (Table 4).
Flock size | Number of dives | Dive duration (s) | Number of pauses | Pause duration (s) | Diving efficiency |
1‒5 | 2354 | 19.9 ± 5.9a | 2249 | 13.2 ± 12.5a | 2.546 ± 1.786a |
6‒10 | 675 | 19.0 ± 5.8b | 638 | 13.0 ± 9.8a | 2.127 ± 1.399b |
11‒15 | 912 | 17.0 ± 5.4c | 869 | 13.0 ± 9.2a | 1.849 ± 1.240c |
16‒19 | 364 | 15.3 ± 5.7d | 348 | 10.7 ± 9.0b | 2.130 ± 1.478b |
In our study, we found that mean dive duration of the Scaly-sided Merganser was shorter than that studied in Yihuang County (23.6 s) (Yihuang County is located in the Fuhe River valley), while mean pause duration was similar to that study (11.6 s) (Shao et al. 2014). The relationship between this difference and water depth, access to food sources, type and availability in the two water areas we investigated requires further study (Benvenuti et al. 2002; Kato et al. 2003; Green et al. 2005; Thompson and Price 2006). Dive durations of the Scaly-sided Merganser were similar to the short time bursts, i.e., 17.5-24.3 s, reported for Barrow's Goldeneyes (Bucephala islandica) (Bourget et al. 2007), Common Goldeneyes (Bucephala clangula) (19.7‒23.9 s) (Bourget et al. 2007) and the Great Crested Grebe (Podiceps cristatus) with 19.5 s (Gomes et al. 2009), as well as that for small diving birds, such as the Little Grebe (Tachybaptus ruficollis) with 13.84 s (Jiang and Shao 2015). These short time bursts were considerably shorter than those reported for large diving birds such as the Subantarctic Cormorant (Phalacrocorax albiventer) with 67‒108 s (Kato et al. 1999), Rock shags (Phalacrocorax magellanicus) with 47.2 s (Quintana 1999) and Red-legged Cormorants (Phalacrocorax gaimardi) with 26.8 s (Frere et al. 2002). Pause durations of the Scaly-sided Merganser were similar to those reported for Barrow's Goldeneyes (12.4‒14.0 s), Common Goldeneyes (10.4‒12.8 s) and the Tufted Duck (Aythya fuligula) (12.96 s) (Halsey et al. 2005). These differences may be related to their body mass, an opinion supported by Cooper's prediction (Cooper 1986).
Diving efficiency (2.297) of our Scaly-sided Merganser was similar to Yihuang section in Fuhe River (1.88‒2.21) while higher than that of the Great Crested Grebe (1.2) (Gomes et al. 2009) and the Little Grebe (1.13) (Jiang and Shao 2015). The American coot (Fulica Americana) enhances its diving efficiency when access to food sources decreases due to prolonged dive duration and shortened pause duration. These differences in diving efficiency may be caused by water depth, light levels and the ecological habitat of the species (Jiang and Shao 2015). Pause duration was positively correlated to earlier dive durations as well as to the duration of the subsequent dive; this agreed with the results obtained by Shao et al. (2014) and those of Jiang and Shao (2015), whose results suggest that the time spent on the surface is a period which the bird uses to recover from the previous dive and to prepare for the next one.
Mean dive duration and mean time on the surface of male Scaly-sided mergansers were significantly longer than those for females and similar to those of Common Goldeneyes (Duncan and Marquiss 1993), the Subantarctic Cormorant, the Japanese Cormorant (Kato et al. 1999) and the Blue-eyed Shag (Laich et al. 2012). These results may be related to body mass differences (Scaly-sided Merganser: W♂: 1.0‒1.2 kg, W♀: 0.8‒1.0 kg; Common Goldeneyes: W♂: 0.8‒1.0 kg, W♀: 0.5‒0.9 kg; Subantarctic Cormorant: W♂: 2.9 kg, W♀: 2.5 kg; Japanese Cormorant: W♂: 3.1 kg, W♀: 2.5 kg; Blue-eyed Shag: W♂ significantly larger than W♀) (Kato et al. 1999; Zhao 2001; Laich et al. 2012) and accord with Cooper's prediction (Cooper 1986).
Dive duration was negatively correlated to the average daily air temperature, which was similar to the results obtained by Green et al. (2005) and may be related to the minimum rate of oxygen consumption. This minimum rate was far smaller in relatively low temperatures than in high temperatures; therefore, with a decrease in the energy cost of diving, the dive duration increased (Green et al. 2005).
Dive duration was negatively related to time of year and daytime. The temperature in December and January were lower than in February and March in our study area. Dive duration was significantly higher in December and January, which may be related to the low minimum rate of oxygen consumption for diving waterfowl under low temperature conditions (Green et al. 2005). However, the temperature in February was lower than in March while dive duration was significantly lower than in March. This unique result, appearing in February, may be caused by increased human activity during China's traditional spring festival. Studies have shown that the diving behavior of waterfowl is limited by light conditions, with dive durations longer at lower light intensity (Wilson et al. 1993; Wanless et al. 1999). Our study supports this general assumption. The longest mean dive duration of the Scaly-sided Merganser was from 7:30‒8:59 when light intensity was low. The dive duration of Scaly-sided Mergansers at 16:00‒17:30 was significantly lower than at any other time, which may be related to their ecological habits or prey behavior.
During this study, we found that the foraging time of our mergansers clearly increased and their vigilance decreased when the flock size was larger, which agrees with the point of view by Roberts (1996) on this issue. That is, with a larger flock, the probability of detecting a predator for individual bird increases while the risk of becoming prey diminishes. That leads to an increase in the foraging time for individual birds and a decrease in the necessity for vigilance (Clark and Mangel 1986). Dive duration of the Scaly-sided Merganser was negatively correlated to flock size, i.e., with a larger flock the dive duration shortens significantly, thereby increasing diving time considerably, quite in agreement with the hypothesis of competitive disturbance. In an environment of limited food sources and larger flocks, individual birds will increase their speed in foraging in order to acquire more food, leading to an increase in forage activity for individual birds and a decrease in vigilance (Clark and Mangel 1986). Furthermore, intraspecific competition was more intense when the group of Scaly-sided Mergansers was larger. In order to acquire more food effectively and decrease intraspecific competition, the Scaly-sided Merganser has adopted a flexible foraging strategy that shortens dive duration and adds to diving times. With an enlargement of flock size, the predation ability and efficiency increases due to collective predation, allowing the Scaly-sided Merganser to acquire more prey in a limited time.
Sex, temperature, time and flock size are factors that affect the diving behavior of our wintering Scaly-sided Merganser. The mean dive duration and mean time on the surface of males were significantly higher than those of females, which may be related to the weight differences between sexes. With an increase in temperatures, dive duration increased considerably, along with the passage of time of year and daytime. The difference of diving behavior at various temperatures and daytime may be related to a low minimum rate of oxygen consumption. With an increase in flock size, dive duration decreased significantly and may be caused by rising interspecific competition.
MQ and BC conceived and designed the experiments. BC performed the experiments and BC and MQ analyzed the data. BC and MQ wrote the paper. Both authors read and approved the final manuscript.
The work was supported by the National Natural Science Foundation of China (Grant No. 31560597).
The authors declare that they have no competing interests.
1. | Luís Eduardo Santos, Liliana de Sousa, Ana Magalhães. Time budget of a mallard duck population residing in an urban park in northern Portugal during courtship and nesting period. acta ethologica, 2024, 27(2): 85. DOI:10.1007/s10211-024-00436-w |
2. | Niujin Shi, Yating Liu, Sanqi Tang, et al. Maximum diving duration and its influencing factors of diving lizards. Behavioral Ecology and Sociobiology, 2024, 78(9) DOI:10.1007/s00265-024-03512-0 |
3. | Peizhong Liu, Meihan Liu, Dongyang Xiao, et al. Scaly-sided Merganser (Mergus squamatus) equalizes foraging costs with depth by switching foraging tactics. Avian Research, 2023, 14: 100129. DOI:10.1016/j.avrs.2023.100129 |
4. | Camille Munday, Paul Rose. Environmental and Social Influences on the Behaviour of Free-Living Mandarin Ducks in Richmond Park. Animals, 2022, 12(19): 2554. DOI:10.3390/ani12192554 |
Sex | Number of focals | Number of dives | Dive duration (s) | Number of pauses | Pause duration (s) | Diving efficiency |
Male | 103 | 2117 | 20.1 ± 6.2a | 2014 | 13.6 ± 12.0a | 2.401 ± 1.732a |
Female | 98 | 2188 | 17.5 ± 5.4b | 2090 | 12.2 ± 10.4b | 2.197 ± 1.510b |
Total | 201 | 4305 | 18.8 ± 6.0 | 4104 | 12.9 ± 11.2 | 2.297 ± 1.626 |
Values with the same letter indicate no significant difference (p > 0.05), while different letters indicate significant differences (p < 0.05), also applying to the tables below |
Temperature (℃) | Number of dives | Dive duration (s) | Number of pauses | Pause duration (s) | Diving efficiency |
4‒10 | 1530 | 21.3 ± 5.5a | 1430 | 12.4 ± 10.1a | 2.579 ± 1.647a |
11‒19 | 1653 | 17.2 ± 5.5b | 1588 | 12.9 ± 11.4a | 2.176 ± 1.670b |
22‒29 | 1122 | 17.7 ± 6.1c | 1086 | 13.5 ± 12.3a | 2.105 ± 1.478b |
Period/daytime | Number of dives | Dive duration (s) | Number of pauses | Pause duration (s) | Diving efficiency |
Month | |||||
Dec. | 842 | 21.1 ± 5.1a | 775 | 13.1 ± 8.2a | 2.136 ± 1.149a |
Jan. | 743 | 21.0 ± 6.3a | 705 | 9.7 ± 10.5b | 3.360 ± 1.985b |
Feb. | 1521 | 16.2 ± 5.3b | 1467 | 13.7 ± 11.2a | 1.903 ± 1.522c |
Mar. | 1199 | 19.1 ± 5.8c | 1157 | 13.7 ± 13.0a | 2.503 ± 1.983d |
Daytime | |||||
7:30‒8:59 | 421 | 19.4 ± 5.3a | 397 | 16.4 ± 13.1a | 1.976 ± 1.555a |
9:00‒15:59 | 3755 | 18.8 ± 6.0a | 3582 | 12.6 ± 11.0b | 2.350 ± 1.646b |
16:00‒17:30 | 129 | 14.9 ± 5.8b | 125 | 11.2 ± 9.8b | 1.811 ± 0.933a |
Flock size | Number of dives | Dive duration (s) | Number of pauses | Pause duration (s) | Diving efficiency |
1‒5 | 2354 | 19.9 ± 5.9a | 2249 | 13.2 ± 12.5a | 2.546 ± 1.786a |
6‒10 | 675 | 19.0 ± 5.8b | 638 | 13.0 ± 9.8a | 2.127 ± 1.399b |
11‒15 | 912 | 17.0 ± 5.4c | 869 | 13.0 ± 9.2a | 1.849 ± 1.240c |
16‒19 | 364 | 15.3 ± 5.7d | 348 | 10.7 ± 9.0b | 2.130 ± 1.478b |