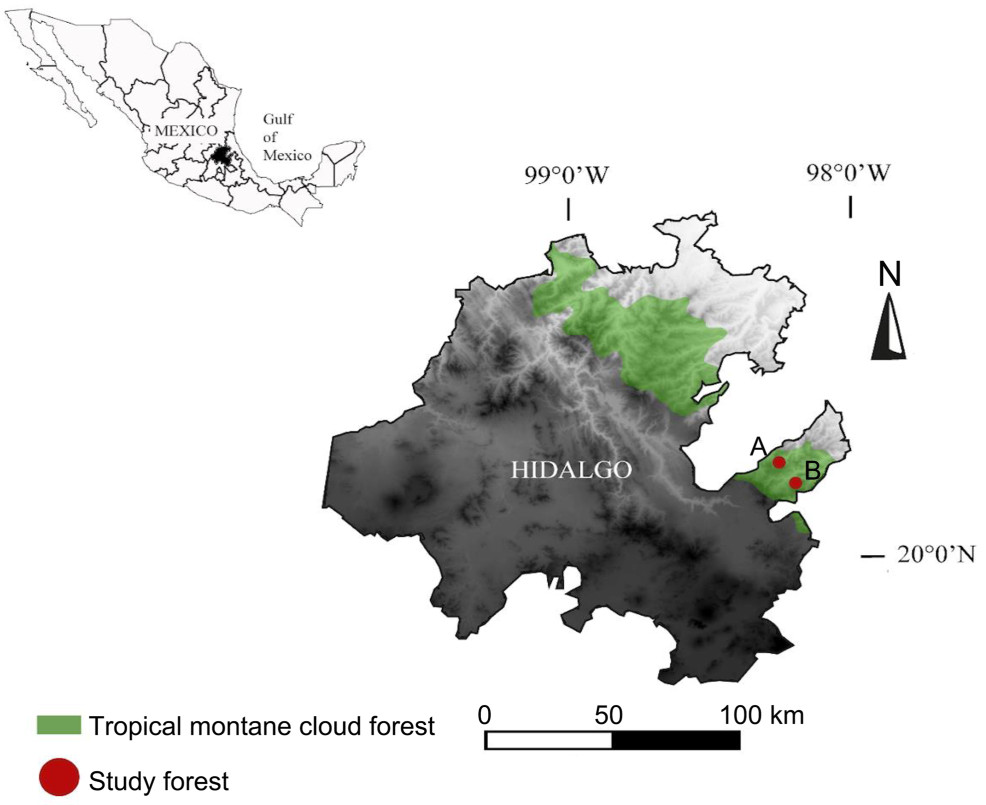
Citation: | Wen Wang, Xiaolong Gao, Sisi Zheng, Zhuoma Lancuo, Ying Li, Lilin Zhu, Jianping Hou, Jiayi Hai, Xin Long, Hanxi Chen, Alexey Druzyaka, Kirill Sharshov. 2021: The gut microbiome and metabolome of Himalayan Griffons (Gyps himalayensis): insights into the adaptation to carrion-feeding habits in avian scavengers. Avian Research, 12(1): 52. DOI: 10.1186/s40657-021-00287-0 |
Himalayan Griffons (Gyps himalayensis), large scavenging raptors widely distributed in Qinghai-Tibetan Plateau, have evolved a remarkable ability to feed on carcasses without suffering any adverse effects. The gut microbiome plays an important role in animal physiological and pathological processes, and has also been found to play a health protective role in the vulture adaptation to scavenging. However, the microbial taxonomic diversity (including nonculturable and culturable microbes), functions, and metabolites related to Himalayan Griffons have not been fully explored.
In the present study, the 28 fecal samples of the Himalayan Griffons and 8 carrion samples were collected and sequenced using high-throughput 16S rRNA gene sequencing methods to analyze the composition and functional structures of the microbiomes. Twelve fecal samples of the Himalayan Griffons were analyzed using untargeted Liquid Chromatography Mass Spectroscopy (LC–MS) to identify metabolites. We used different culture conditions to grow Himalayan Griffons gut microbes. Inhibitory effects of gut beneficial bacteria on 5 common pathogenic bacteria were also tested using the Oxford cup method.
According to the results of the culture-independent method, a high abundance of four major phyla in Himalayan Griffons were identified, including Fusobacteria, Firmicutes, Bacteroidetes, and Proteobacteria. The most abundant genera were Fusobacterium, followed by Clostridium_sensu_stricto_1, Cetobacterium, Epulopiscium, and Bacteroides. The predicted primary functional categories of the Himalayan Griffons' gut microbiome were associated with carbohydrate and amino acid metabolism, replication and repair, and membrane transport. LC–MS metabolomic analysis showed a total of 154 metabolites in all the fecal samples. Cultivation yielded 184 bacterial isolates with Escherichia coli, Enterococcus faecium, Enterococcus hirae, and Paeniclostridium sordellii as most common isolates. Moreover, 7 potential beneficial gut bacteria isolated showed certain inhibition to 5 common pathogenic bacteria.
Our findings broaden and deepen the understanding of Himalayan Griffons' gut microbiome, and highlighted the importance of gut microbiome-mediated adaptation to scavenging habits. In particular, our results highlighted the protective role of gut beneficial bacteria in the Himalayan Griffons against pathogenic bacteria that appear in rotten food resources.
Tropical montane cloud forests (TMCFs) are one of the most important hotspots on Earth due to the presence of relict-endemic and endangered species (Ponce-Reyes et al. 2012; Hu and Riveros-Iregui 2016; Rahbek et al. 2019). TMCF represent about 14% of the total tropical forest worldwide (Mulligan 2010), principally found in Asia, North and South American and Africa, comprising varied structures, floristic affinities, and very diverse species composition (Roman et al. 2010; Toledo-Aceves et al. 2011). This type of vegetation is characterized by the frequent presence of fog and/or drizzle causes persistent moisture (60–85%) throughout the year (Gual-Díaz and Rendón-Correa 2014), and usually thrives on steep slope ravines (> 30°) with specific microenvironmental features (e.g. moisture, soil type, pH, among others) (Rodríguez-Ramírez et al.2018a). The Mexican TMCF shows a high diversity of tree species (e.g. Liquidambar, Quercus, Fagus, Magnolia, Pinus, Podocarpus, Clethra, Meliosma, Tilia, Carpinus, Nyssa, Ostrya, Acer, Alnus, Symplocos and Prunus; Luna-Vega et al. 2000; Toledo-Aceves et al. 2011); unfortunately, it currently covers < 1%, and faces a drastic reduction in area (over 50%) caused by logging to clear areas for pasture, crop fields or avocado, corn and/or coffee plantations (Muñoz-Villers and López-Blanco 2008; Gual-Díaz and Rendón-Correa 2014).
The TMFC has a pronounced seasonality (e.g. fog, rainfall, moisture and temperature) that influences phenological patterns such as seed production or masting (Kelly 1994; Pearse et al. 2016) which in turn affects seed dispersal/or predation by mammals and birds (Jensen 1985). Nevertheless, drastic climate fluctuations, habitat fragmentation and isolation create unprecedented pressures, causing irregular seed production (Godínez-Ibarra et al. 2007).
Birds are dispersal agents that are a key in the functionality and process (i.e. synchronic seed production) of forest ecosystems (Jansen et al. 2004; Amico and Aizen 2005; Zaccagnini et al. 2011; Fletcher 2015) by influencing population dynamics, social structure and disease prevalence (Martínez-Morales 2007; Navarro-Sigüenza et al. 2014). Different bird species, notwithstanding, respond negatively or positively to fragmentation, anthropic activities and/or land-use, affecting ecosystem networks (Martínez-Morales 2005; Hagen et al. 2012; Rueda-Hernandez et al. 2015).
In the TMCFs, avian communities occur with a high degree of habitat specialization and many species have low dispersal capacity, making this a habitat of ecological importance for avian conservation (e.g. Dendrortyx barbatus, Zentrygon albifacies, Cyanolyca nana; Martínez-Morales 2007). Studies of Mexican TMCF avian communities have found contrasting species richness and high species turnover among fragments (Rueda-Hernandez et al. 2015, state of Veracruz; Calderón-Patrón et al. 2016, state of Hidalgo). Another study in Mexican Beech forests in Hidalgo (a vegetation type associated with the TMCF) reported that during masting, several seed bird consumers were observed (Ehnis 1981). Nevertheless, it is necessary to explore how avian structure (e.g. richness, abundance and evenness) and composition (e.g. identity of species) respond to changes in plant associations with restricted distribution and dramatic seed production behavior through masting year (i.e. synchronic seed production and non-seed production).
Mexican Beech (Fagus grandifolia subsp. mexicana (Martínez) A. E. Murray) is a relict-endemic tree species, which is dominant and a common representative of small TMCF fragments (1‒42.5 ha) in the Sierra Madre Oriental in eastern Mexico found between 1400 and 2000 m a.s.l., mainly on ravine slopes (43.8°) (Williams-Linera et al. 2003; Téllez-Valdés et al. 2006). Mexican Beech is characterized by synchronic seed production (at 2- to 8-year intervals; Ehnis 1981; Rodríguez-Ramírez et al. 2018a). This strategy favors the fitness of plants and economizes the cost of reproduction (the predator satiation hypothesis; Bogdziewicz et al. 2020).
Fagus is a genus distributed in North America, Europe and Asia. Several studies associated with specific beech forests [e.g.Fagus grandifolia Ehrn in USA (Darley-Hill and Johnson 1981; Rosemier and Flaspohler 2006), F. sylvatica L. in Europe (Perdeck et al. 2000; Kardell 2005; Chamberlain et al. 2007), and F. crenata Blume in Japan (Yui 1991; Yasaka et al. 2003)] reported that beechnuts are an important resource of the diet of several bird species. Meanwhile, the studies emphasize the importance of local (e.g. vegetation structure, anthropic activities) and landscape-scale (e.g. fragmentation, edge effect) variables as factors that negatively influence bird species richness (Martínez-Morales 2005; Wells et al. 2011).
In Europe, high avian turnover was associated with the Common Beech (F. sylvatica) related forest attributes and microclimatic conditions (Mentil et al. 2018). In addition, Grendelmeier et al. (2019) found that the mast seeding in the Common Beech may influence bird abundance directly (seed consumers) and indirectly (non-seed consumers), which affects ecological interactions.
In this study, we provided the first assessment of alpha and beta avian diversity (during a masting year, 2017) and how bird diversity and the composition of trophic guilds modifies during three phenological processes: (1) immature seeds (IS; June‒July); (2) mature seeds (MS; August); and (3) low seed quality (LQ; September) in two fragmented Mexican Beech forests. Therefore, we hypothesized that the phenological process given for the changes during the masting event and between the study forests by contrasting degree of fragmentation may affect the alpha, beta avian diversity, and the composition of trophic guilds. Furthermore, according to Martínez-Morales (2007), bird communities are still unexplored in our study forests, so we provide valuable new information. In addition, we estimated beechnut production during a masting event and compared between fragmented forests to complement the characterization of the phenological process of this relict-endemic type of vegetation.
We studied two isolated and fragmented Mexican Beech forests in the state of Hidalgo (Fig. 1) which are considered the largest and genetically heterogeneous beech forests in Mexico, according to Pérez-Rodríguez (1999). This type of vegetation occurs under restricted climatic conditions within the TMCF, located in the Sierra Madre Oriental (Téllez-Valdés et al. 2006). Our two localities were separated by 15.58 km and presented the following characteristics:
1) The locality of Medio Monte in the municipality of San Bartolo Tutotepec (20°24′50″ N, 98°14′24″ W at 1800–1944 m a.s.l.) is one of least disturbed and largest fragment Mexican Beech forest (34.25 ha), located in the northwestern part of the municipality surrounded by steep montane ravines. This study forest has a temperate climate (Cwb; Kottek et al. 2006; Peel et al. 2007) with mild temperatures (14.5 to 24.2 ℃) and annual precipitation between 1200 and 2000 mm (Rodríguez-Ramírez et al. 2018b). Medio Monte is characterized by trees reaching up to 25 m in height, with a canopy dominated by Mexican Beech, Patula Pine (Pinus patula Schltdl. & Cham.), Mountain Magnolia or Yoloxóchitl (Magnolia schiedeana Schltdl.) and Red Oak (Quercus meavei S. Valencia-A., Sabás & O.J. Soto). The mid-canopy is composed of Mexican Clethra (Clethra mexicana DC.), White Oaks (Q. delgadoana S. Valencia, Nixon & L.M. Kelly, Q. trinitatis Trel.), whereas the low canopy consists mainly of two tree ferns species: Cyathea fulva (M. Martens & Galeotti) Fée and Dicksonia sellowiana var. arachneosa Sodiro (Rodríguez-Ramírez et al. 2018b).
2) The locality of El Gosco in the municipality of Tenango de Doria (20°19′37.8″ N, 98°14′57.1″ W at 1557–1864 m a.s.l.) is the smallest and most fragmented Mexican Beech forest (4.5 ha) located in the western part of the municipality surrounded by montane slope ravines. This study forest has been mainly disturbed by illegal logging (Rodríguez-Ramírez et al. 2013). The climate is temperate (Cwb; Kottek et al. 2006; Peel et al. 2007) with an average annual temperature of 17 ℃ and an annual precipitation of 1733 mm with < 60 mm rainfall rates per month (Rodríguez-Ramírez et al. 2019). In the study forest, the high canopy (≥ 20 m) is composed of Mexican Beech, Patula Pine, White Oak, Sweetgum (Liquidambar styraciflua L.), Tarflower (Befaria aestuans L.), and Mountain Magnolia. The mid-canopy (10 to 20 m) is dominated by Neotropical tree species, such as Zapotillo (Sideroxylon portoricense subsp. minutiflorum (Pittier) T.D. Penn.), Sabino (Podocarpus reichei J. T. Buchholz & N.E. Gray), Sweetwood (Nectandra salicifolia (Kunth) Nees) and Wild Avocado (Beilschmiedia mexicana (Mez) Kosterm) (Rodríguez-Ramírez et al. 2018b).
We characterized and described the three phenological events in each study forest by assessing the beechnut production in three phenological processes: immature seeds (IS), empty or contains an undeveloped embryo; mature seeds (MS), presence of well-developed embryo; and low seed quality (LQ), presence of empty seeds or infested by fungi (Fig. 2). In each process, we collected, counted and classified beechnuts monthly in four categories: (1) undamaged; (2) attacked by insects (i.e. typical oviposition marks or with larvae); (3) empty (by the endosperm absence); and (4) immature (according to Godínez-Ibarra et al. 2007). The beechnuts or seeds (1.5 cm in length) are involved by a spiny, four-valvate cupule (Rodríguez-Ramírez et al. 2016).
We selected 30 mature Mexican dominant beech trees (i.e. with a diameter at breast height ≥ 40 cm at 1.5 m from the trunk (Rodríguez-Ramírez et al. 2018b) in each study forest. The distance between individual trees was approximately 10 m, which is far enough to ensure seed production data independence (Rodríguez-Ramírez et al. 2018b). After mass flowering began (May), we placed 30 circular seed traps in each study forest. Each trap (1 m2 in surface area) consisted of a plastic funnel that was attached to a PVC tube with a diameter of 5.08 cm and a height of 1 m to avoid seed removal by mammals and birds (the main predators in the study forests) after seed drop. The beechnut data obtained from the traps were reported by percentage.
At each study forest, we carried out one survey during each phenological process (from June to September) in a masting year (2017). Each study forest was visited once in the morning (1 h after dawn) and once in the evening (2 h before sunset) to standardize the daily avian species fluctuation to guarantee a high completeness of inventory. To register avian diversity exclusive to the Mexican Beech forest, we followed two complementary survey methods (Olvera-Vital et al. 2020) due to distinctive features of sites, like steep-slope montane ravines and closed canopy (> 90%; Rodríguez-Ramírez et al. 2013):
1) We located seven transects (250 m × 10 m) in each fragmented forest, which walked slowly for 10 to 15 min, and all birds seen or heard were recorded. 2) Also, seven 25 m-radius point counts were located at each forest; minimum distance from point to point was 250 m to ensure data independence, and birds were recorded for 15 min (modified by Ralph et al. 1996). We used avian field guides for identifying species (Peterson and Edward 1989; Howell and Webb 1995; National Geographic Society 2006; van Perlo 2006). The residence status of avian species was classified according to Martínez-Morales (2007), and trophic guilds were based on foraging behavior (Howell and Webb 1995; Billerman et al. 2020) as well as personal observations during fieldwork.
We used the sample coverage estimator suggested by Chao and Jost (2012), with values from 0 (minimal completeness) to 100% (maximum completeness) to evaluate avian survey effort considering individuals. Alpha avian diversity was calculated using the indices q = 0 that represents species richness, and q = 1 which represents the exponential value of the Shannon index that considers species and their abundances (ecological diversity; Hill 1973). These analyses were performed among three phenological processes and between fragmented forests.
For a given diversity order q, the alpha diversity is:
qD=(S∑i=1pqi)1/(1−q) |
where S is the number of species, pi is the relative abundance of species i, and q is the order number of diversity. Different values of q correspond to different ecological diversity indices; q = 0 corresponds to species richness, which places the maximum weight on rare species (abundances are ignored), and q = 1 corresponds to algebraic transformations of the Shannon index of diversity. As q increases, the Hill number index is increasingly weighted by the relative abundances of common species (Jost 2006). These values were compared using 95% confidence intervals. We used the iNEXT R library (Chao et al. 2014; R Core Team 2018) to compute the analytical solutions for Hill's numbers q = 0 and 1.
We used rank-abundance curves to assess the structure of avian assemblages and to compare among three phenological processes and between fragmented forests (Magurran 2004). We also compared differences in avian composition among three phenological processes (IS, MS and LQ) and between fragmented forests (Medio Monte and El Gosco) by building Bray–Curtis similarity matrices. We performed a PERMANOVA analysis to assess the similarity of residual values after 999 permutations under the reduced model (Clarke 1993). We performed a non-metric multidimensional scaling (NMDS) to show avian compositional differences and how they are modified through phenological process and between fragmented forests. NMDS ordinations were based on Bray–Curtis distances and 30 randomizations to determine the most stable solution. We used the stress-plot function and the Stress index to assess the values of the ordination. These analyses were performed in R software using the R-package vegan (Oksanen et al.2019).
We calculated the complementarity index described by Colwell and Coddington (1994). The index varies from zero (when the lists are identical) to unity (when the lists are completely distinct); also, could be expressed as the percentage of species from 0 to l00%. To represent shared and exclusive avian species among three phenological processes and between fragmented forests were performed Venn diagrams.
We partitioned beta diversity following the procedure of Podani and Schemera (2011) based on the approach of Baselga (2010). According to this method, total dissimilarity (βcc) is 1 minus the similarity coefficient of Jaccard. The βcc is divided into two components: turnover (β.3, replacement of one species by another) and nestedness (βrich, differences in species richness). This partition was developed for analyzing the dissimilarity in avian composition between phenological process (IS and MS, MS and LQ, IS and LQ) and between fragmented forests (Medio Monte and El Gosco). These analyses were performed in R software (R Core Team 2018), using the R-script of Carvalho et al.(2012, 2013).
We found empty and immature beechnuts (100%) during IS in both fragmented forests. During MS, Medio Monte registered mostly undamaged seeds (85%) and only 15% were empty, while El Gosco registered a large amount of empty seeds (89%), a few seeds attacked by insects (9%) and a low proportion of seeds were immature (2%). During LQ, both forests registered empty seeds (48% for Medio Monte and 20% for El Gosco), while the rest were infested by fungi (Fig. 3).
We registered 36 species from 20 families and 33 genera (Table 1). Of them, 83% were residents, 8% presented resident and migratory populations, 6% were classified as migratory and 3% were transient; we did not determine the status of one individual that was not identified at the species level (Table 1). Eleven species are included in some threatened status by Mexican laws (SEMARNAT 2010; Table 1). Five species are endemic to Mexico and one is restricted to Mexico, the United States and Canada (Martínez-Morales 2007) (Table 1).
Family | Species | Trophic group | IS | MS | LQ | Medio Monte (N) | El Gosco (N) | Residence status |
Odontophoridae | Dendrortyx barbatus a* | G, F, I | X | X | 2 | 1 | R | |
Columbidae | Zentrygon albifacies** | G, F | X | 7 | 4 | R | ||
Trochilidae | Lampornis amethystinus | N | X | 211 | 0 | R | ||
Lamprolaima rhami* | N | X | 0 | 2 | R | |||
Amazilia cyanocephala | N | X | 0 | 2 | R | |||
Hylocharis leucotis | N | X | 0 | 4 | R | |||
Strigidae | Aegolius acadicus | C | X | 3 | 0 | R | ||
Trogonidae | Trogon caligatus | F, I | X | 0 | 1 | R | ||
Trogon mexicanus | F, I | X | X | X | 26 | 6 | R | |
Ramphastidae | Aulacorhynchus prasinus*** | F, I | X | 4 | 2 | R | ||
Picidae | Melanerpes formicivorus | F, I | X | X | X | 23 | 2 | R |
Picoides fumigatus | I | X | X | 122 | 1 | R | ||
Colaptes auratus | I | X | 0 | 1 | R | |||
Psittacidae | Pionus senilisa** | G, F | X | 12 | 0 | R | ||
Furnariidae | Sclerurus mexicanus** | I | X | 2 | 0 | R | ||
Xiphorhynchus erythropygius** | I | X | X | 0 | 2 | R | ||
Tyrannidae | Empidonax sp. | X | 0 | 1 | - | |||
Empidonax occidentalisb | I | X | X | 1 | 1 | R | ||
Empidonax fulvifrons | I | X | 1 | 0 | R | |||
Vireonidae | Vireo leucophrys | I | X | 1 | 1 | R | ||
Corvidae | Cyanolyca nanaa* | I | X | 4 | 0 | R | ||
Certhiidae | Certhia americanaa* | I | X | 3 | 0 | R | ||
Throglodytidae | Troglodytes aedon | I | X | 1 | 0 | R, M | ||
Hernicorhina leucophrys | I | X | 2 | 0 | R | |||
Polioptilidae | Poliotila caerulea | I | X | 2 | 0 | R, M | ||
Turdidae | Myadestes occidentalis*** | G, I, F | X | X | X | 33 | 11 | R |
Catharus aurantiirostris | G, I, F | X | 2 | 0 | R | |||
Catharus guttatus | O | X | X | 2 | 2 | M | ||
Turdus migratorius | F, I | X | X | 6 | 1 | R, M | ||
Mimidae | Melanotis caerulescensa | O | X | 2 | 0 | R | ||
Fringillidae | Euphonia elegantissima | F | X | 1 | 0 | R | ||
Parulidae | Setophaga dominica | I | X | 1 | 0 | M | ||
Basileuterus belli | I | X | 3 | 0 | R | |||
Myioborus pictus | I | X | 1 | 22 | R | |||
Cardinalidae | Piranga leucoptera | F, I | X | X | 0 | 3 | R | |
Habia fuscicauda | G, I, F | X | 1 | 0 | R | |||
Passerina ciris*** | G, I, F | X | 2 | 0 | T | |||
IS, immature seeds; MS, mature seeds; and LQ, low seed quality; Presence (X) and relative abundance (N) are reported. Bird nomenclature follows the American Ornithological Society (Chesser et al. 2019). Trophic guild: F, frugivore; G, granivore; I, insectivore; N, nectarivore; O, omnivore; C, carnivore. Residence status: R, breeding resident; M, winter migrant; T, transient Endemism: a endemic to Mexico and b restricted distribution Endangered species according to NOM-059 (SEMARNAT 2010) * Endangered; **Threatened; and *** Special Protection |
All bird species registered in each fragmented forest were previously reported for the state of Hidalgo (Martínez-Morales 2007). Also, we confirmed the presence of the Garnet-throated Hummingbird (Lamprolaima rhami), previously considered hypothetical for Hidalgo, and we report the first record of the Northern Saw-whet Owl (Aegolius acadicus) in the TMCF in Hidalgo, previously reported for oak and pine forests (Martínez-Morales 2007). The bird species represent nine trophic guilds: 42% of the species are insectivores, 16% frugivores-insectivores, 11% granivores-insectivores-frugivores, 11% nectarivores, 5% granivores-frugivores, 5% omnivores, 3% frugivores, 3% carnivores, and 3% granivores-frugivores-insectivores (Table 1).
Avian communities among three phenological processes were structurally similar, dominated by few bird species. For example, during IS the Brown-backed Solitaire (Myadestes occidentalis, granivores-insectivores-frugivores), the Mountain Trogon (Trogon mexicanus, frugivores-insectivores) and the Acorn Woodpecker (Melanerpes formicivorus, frugivores-insectivores) were the most abundant avian species, while the White-crowned Parrot (Pionus senilis, granivores-frugivores), the White-faced Quail-Dove (Zentrygon albifacies, granivores-frugivores) and the Brown-backed Solitaire were abundant during MS, and the Dwarf Jay (Cyanolyca nana, insectivores) was abundant during LQ process (Fig. 4a; Table 1). We found a similar pattern between fragmented forests, where the Brown-backed Solitaire and Mountain Trogon were the most abundant (Fig. 4b).
The completeness of the avian inventory was greater than 80% in the three phenological processes and between fragmented forests (Table 2). Higher avian richness was recorded during IS followed by MS and the lowest richness during the LQ process (q = 0; Fig. 5a). Medio Monte showed a higher avian richness than El Gosco (q = 0; Fig. 5b; Table 2). Ecological diversity (richness and abundance, q = 1) did not differ among three phenological processes (Fig. 5c; Table 2) and between fragmented forests (q = 1; Fig. 5d). Our PERMANOVA analysis showed significant differences in avian communities' composition among three phenological processes (pseudo-F = 3.26, df = 2, P = 0.05) and no differences between fragmented forests (pseudo-F = 1.64, df = 1, P = 0.24) were reflected in the NMDS ordination (Fig. 6).
q=0 | Cl | q=1 | Cl | Sc | |
Study forest | |||||
El Gosco | 19 | 3.48 | 13.8 | 3.52 | 0.83 |
Medio Monte | 29 | 3.53 | 14.11 | 2.64 | 0.94 |
Phenological process | |||||
IS | 24 | 4.07 | 10.09 | 2.39 | 0.93 |
MS | 15 | 1.71 | 10.16 | 1.2 | 0.88 |
LQ | 11 | 2.36 | 10 | 2.33 | 0.82 |
El Gosco | |||||
IS | 10 | 2.81 | 7.67 | 2.7 | 0.8 |
MS | 7 | 1.93 | 6.3 | 1.7 | 0.88 |
LQ | 7 | 1.97 | 6.61 | 2.11 | 0.49 |
IS | 10 | 7.67 | 7.67 | 2.7 | 0.8 |
MS | 7 | 6.3 | 6.3 | 1.7 | 0.88 |
Medio Monte | |||||
IS | 17 | 2.81 | 7.89 | 1.68 | 0.96 |
MS | 13 | 3.8 | 8.99 | 2.73 | 0.86 |
LQ | 7 | 2.76 | 5.85 | 3.02 | 0.56 |
IS | 17 | 2.81 | 7.89 | 1.68 | 0.96 |
MS | 13 | 3.8 | 8.99 | 2.73 | 0.86 |
IS, immature seeds; MS, mature seeds; and LQ, low seed quality |
The shared complementarity index value was 86.6% between IS and MS, 92.3% between MS and LQ, and 90.9% between IS and LQ. We detected 15 exclusive avian species during IS, 7 species during MS, and 5 species during LQ (Fig. 7a). Between fragmented forests, the shared index value was 56%, and 18 species were exclusive to Medio Monte and 8 to El Gosco (Fig. 7b).
The total average avian beta diversity (βcc = 0.78) between IS and MS was mainly explained by turnover (β.3 = 0.5) and followed by nestedness in a low proportion (βrich = 0.28; Fig. 8a). The same pattern existed between MS and LQ, where the total average beta was 0.81, with high turnover (β.3 = 0.63) and low nestedness (βrich = 0.18; Fig. 8a). Between IS and LQ, the total average beta was 0.83, and the turnover and nestedness had similar values (β.3 = 0.40, and βrich = 0.43; Fig. 8a).
The total average avian beta diversity between fragmented forests (βcc = 0.70) was mainly explained by turnover (β.3 = 0.43) and followed by nestedness in a low proportion (βrich = 0.27; Fig. 8b). Beta diversity during the LQ process was not assessed within each fragmented forest due to the limited data recorded (Table 2).
Overall, our results showed that the phenological process influenced alpha and beta avian diversity values. Differences were also observed between the two fragmented forests. Alpha diversity showed differences in avian species richness (q = 0) among three phenological processes. During IS a higher species richness was registered, represented by dominant insectivorous-frugivorous species (e.g. Myadestes occidentalis, Trogon mexicanus, and Melanerpes formicivorus). In this process, beechnuts were immature or attacked by insects, and it is possible that these resources attracted those insectivorous birds that feed on them. Later, during the MS we observed dominance by granivorous-frugivorous species (e.g.Zentrygon albifacies and Pionus senilis) and the beechnuts were undamaged; probably the masting period influenced the presence of seed bird consumers and forced non-seed consumers to search for other resources. In the LQ process was registered lower species richness, dominated again by insectivorous species (i.e. Cyanolyca nana), and during this process, seeds were empty, so possibly the presence of insects became the most important resource.
When comparing fragmented forests, we found that the bigger, least-disturbed and better-connected fragment showed highest undamaged beechnut production (Medio Monte), which also showed a higher avian species richness than the severely fragmented forest (El Gosco) with a very low seed production. Previous study in the TMCF identified that the size of the fragment was the most important feature having a positive relationship with species richness; while the connectivity did not seem to influence the bird species richness (Martínez-Morales 2005). Bird abundance patterns were similar among the three phenological processes and between forests, thus the beechnut presence had no influence on the number of bird individuals, a possible explanation could be related with found it by Martínez-Morales (2007) where generally the abundance distribution of bird community in the TMCF is given by a few bird species were very abundant and many species with scarce abundance, this pattern was reflected in the Fig. 4 where few bird species were dominant through phenological processes and between forests. However, other particular forest fragments features are needed to measure and evaluate the specific requirements of bird community, such as roosting and shelter sites, feeding, nesting and protection from predators could be influenced our results (Martínez-Morales 2005). Further studies related with the bird species life-traits and specific measurements of microenviromental features in the Mexican Beech forests are necessary.
Our field observations confirm that the granivorous-frugivorous White-faced Quail-Dove and the White-crowned Parrot are potential Mexican beechnut-dispersal agents and/or predators. This is consistent with similar results reported by (Ehnis 1981) who addresses that parrots and other bird species visit the Mexican Beech forests only during masting. Several studies in American Beechnut (Fagus grandifolia) and Common Beechnut have highlighted the relevance of seed bird consumers, and their direct influence as dispersal and/or predators in the regeneration of those forests; for example, the now-extinct Passenger Pigeon (Ectopistes migratorius (Guiry et al. 2020), the Nutcracker (Nucifraga caryocatactes), the Blue Jay (Cyanocytta cristata), the Mallard (Anas platyrhynchos), the Common Wood Pigeon (Columba palumbus) and the Eurasian Jay (Garrulus glandarius) (Turcek and Kelso 1968; Darley-Hill and Johnson 1981). On the other hand, the Brambling (Fringilla montifringilla), the Eurasian Nuthatch (Sitta europaea), and the Marsh Tit (Poecile palustris) have been reported as short-distance dispersal agents (Nilsson 1985). During a masting event the Mexican Beech forest features trigger different responses for seed and non-seed bird consumers. For example, granivorous and frugivorous bird species are directly benefited, while insectivorous bird species could benefit indirectly by the presence of arthropods (i.e. insects, myriapods and arachnids; Wells et al. 2011), as well as other specific requirements of certain bird species, such as roosting areas, nesting grounds, and protection from predators (Martínez-Morales 2007).
Our results reflect the importance of the TMCFs and their tree communities (i.e. Mexican Beech forests) as refuges ("safe sites"; Reid 1991) for maintaining several endemic, threatened, and range-restricted bird species, as previous studies have established (Martínez-Morales 2005, 2007; Navarro-Sigüenza et al. 2014; Rueda-Hernandez et al. 2015). For example, the Bearded Wood-Partridge (Dendrortyx barbatus), the White-faced Quail-Dove, the Tawny-throated Leaftosser (Sclerurus mexicanus), the Spotted Woodcreeper (Xiphorhynchus erythropygius), the Brown-capped Vireo (Vireo leucophrys), the Dwarf Jay, the Grey-breasted Wood-Wren (Henicorhina leucophrys), and the Golden-browed Warbler (Basileuterus belli) are considered to be restricted to TMCF (Martínez-Morales 2005; Sánchez-González and Navarro-Sigüenza 2009).
In our study, we found a high bird species complementarity between phenological processes. For example, the highest value was between the MS‒LQ, where only one exclusive species belonged to the granivorous-frugivorous-insectivorous guilds, followed by the IS‒LQ process where two exclusive bird species belonged to the insectivorous and fructivorous-insectivorous guilds. The lowest value was between the IS‒MS process, where four exclusive bird species belonged to the insectivorous, omnivorous, and fructivorous-insectivorous guilds. Between phenological processes a low interspecific competition can be expected, because food resources are better diversified (e.g. seeds and insects; Loreau 2000). Between fragmented forests, however, we found a medium bird species complementarity value where the exclusive species were represented by different trophic guilds; possibly increasing the interspecific competition by the limited food resources adding the contrast features between forests (e.g. logging, fragment size, isolation, and complex structure vegetation).
High turnover was detected in the transition between IS‒MS and between MS‒LQ, as well as between fragmented forests. These results are possibly related by the forest attributes (i.e. vegetation structure, topography, drizzle and/or fog) triggered that beechnuts production impacting the food resources available for some specific bird species (i.e. Zentrygon albifacies and Pionus senilis). Previously, Rueda-Hernandez et al. (2015) in the state of Veracruz, Mexico, Calderón-Patrón et al. (2016) in the state of Hidalgo, and Rahbek et al. (2019) worldwide reported a high avian turnover in the TMCFs, which was related to habitat heterogeneity. Meanwhile, in Europe the high avian turnover was associated with the Common Beech forest attributes and microclimatic conditions (Mentil et al. 2018).
Nevertheless, we found similar values of beta diversity components (turnover and nestedness) between IS‒LQ showing that masting influenced a high dissimilarity in the avian community, but beechnut absence allowed both components of beta diversity to equally affect these assemblages. This phenomenon is related to the predator satiation hypothesis (Kelly 1994), which states that the presence of synchronous masting (seed production) will maximize the probability of satiating the seed consumer predators, allowing some seeds to germinate. Likewise, masting could influence avian species replacement, even though the responses depend on factors like mobility, life history and diet of seed predators (Bogdziewicz et al.2020). Therefore, our results supported the hypothesis regarding phenological process (IS, MS and LQ) influencing alpha and beta avian diversity and their trophic guilds composition during masting events, as well as in two contrasting Mexican Beech forests.
Particularly, inter-annual variation in plant reproduction (masting) of relict-endangered Mexican Beech forest depends on specific climatic conditions (e.g. temperature and/or precipitation; Rodríguez-Ramírez et al. 2018a, b). However, other studies (Téllez-Valdés et al. 2006) showed that climatic fluctuations and anthropogenic landscape changes (e.g. grazing, cattle, agricultural activity and mixed crops; Rodríguez-Ramírez et al. 2013) have possibly modified the synchronous process by an asynchronous one (Burns 2012) directly influencing the seed bird consumers and, indirectly, the non-seed bird consumers (Grendelmeier et al. 2019) modifying the ecological interaction within forests. Future studies are necessary, for example to: (1) assessing ex situ the effect of White-faced Quail-Dove, White-crowned Parrot or other resident bird species can be considered beechnut dispersers, seed consumers or non-seed consumers; (2) evaluate beechnut quality among Mexican Beech fragments; and (3) relate bird diversity with masting years versus non-masting year.
Our results are the first evidence that shows that masting phenological process influences the bird species richness and turnover in relict-endangered Mexican Beech forests, but not abundance patterns, the same tendency was found in contrasting Mexican Beech fragmented forests. In accordance to Rodríguez-Ramírez et al. (2013) and Téllez-Valdés et al. (2006), Mexican Beech forests are a good model to identify priority areas for conservation in the TMCF, due to its restricted distribution and high biological value (e.g. high species richness, endemisms and presence of endangered species). Finally, it is imperative to address regional conservation efforts to preserve this threatened ecosystem due to constant anthropogenic pressure.
We wish to thank I. Zuria for her valuable comments to improve this manu- script. We thank Mary-Ann Hall and I. Zuria for checking the language of this manuscript.
ECR-R: conceptualization, methodology, investigation, writing, review and editing. LC-I, APM-F: methodology, writing, review and editing. IL-V: writing, review and editing. PC-R: conceptualization, supervision, methodology, writ- ing, review and editing. All authors read and approved the final manuscript.
The datasets used in the present study are available from the corresponding author on reasonable request.
Not applicable.
Not applicable.
The authors declare that they have no competing interests.
Barnes A, Kaur A, Augenbraun M. An unusual presentation of prostatic abscess due to Actinomyces turicensis and Peptostreptococcus. Cureus. 2020;12: e8665.
|
Lee WY. Avian gut microbiota and behavioral studies. Korean J Ornithol. 2015;22: 1-11.
|
Mateos-Hernández L, Crespo E, de la Fuente J, de la Lastra JMP. Identification of key molecules involved in the protection of vultures against pathogens and toxins. In: Radis-Baptista G, editor. An integrated view of the molecular recognition and toxinology: from analytical procedures to biomedical applications. Rijeka: InTech; 2013. p. 241-65.
|
Waite DW, Taylor MW. Characterizing the avian gut microbiota: membership, driving influences, and potential function. Front Microbiol. 2014;5: 223.
|
Family | Species | Trophic group | IS | MS | LQ | Medio Monte (N) | El Gosco (N) | Residence status |
Odontophoridae | Dendrortyx barbatus a* | G, F, I | X | X | 2 | 1 | R | |
Columbidae | Zentrygon albifacies** | G, F | X | 7 | 4 | R | ||
Trochilidae | Lampornis amethystinus | N | X | 211 | 0 | R | ||
Lamprolaima rhami* | N | X | 0 | 2 | R | |||
Amazilia cyanocephala | N | X | 0 | 2 | R | |||
Hylocharis leucotis | N | X | 0 | 4 | R | |||
Strigidae | Aegolius acadicus | C | X | 3 | 0 | R | ||
Trogonidae | Trogon caligatus | F, I | X | 0 | 1 | R | ||
Trogon mexicanus | F, I | X | X | X | 26 | 6 | R | |
Ramphastidae | Aulacorhynchus prasinus*** | F, I | X | 4 | 2 | R | ||
Picidae | Melanerpes formicivorus | F, I | X | X | X | 23 | 2 | R |
Picoides fumigatus | I | X | X | 122 | 1 | R | ||
Colaptes auratus | I | X | 0 | 1 | R | |||
Psittacidae | Pionus senilisa** | G, F | X | 12 | 0 | R | ||
Furnariidae | Sclerurus mexicanus** | I | X | 2 | 0 | R | ||
Xiphorhynchus erythropygius** | I | X | X | 0 | 2 | R | ||
Tyrannidae | Empidonax sp. | X | 0 | 1 | - | |||
Empidonax occidentalisb | I | X | X | 1 | 1 | R | ||
Empidonax fulvifrons | I | X | 1 | 0 | R | |||
Vireonidae | Vireo leucophrys | I | X | 1 | 1 | R | ||
Corvidae | Cyanolyca nanaa* | I | X | 4 | 0 | R | ||
Certhiidae | Certhia americanaa* | I | X | 3 | 0 | R | ||
Throglodytidae | Troglodytes aedon | I | X | 1 | 0 | R, M | ||
Hernicorhina leucophrys | I | X | 2 | 0 | R | |||
Polioptilidae | Poliotila caerulea | I | X | 2 | 0 | R, M | ||
Turdidae | Myadestes occidentalis*** | G, I, F | X | X | X | 33 | 11 | R |
Catharus aurantiirostris | G, I, F | X | 2 | 0 | R | |||
Catharus guttatus | O | X | X | 2 | 2 | M | ||
Turdus migratorius | F, I | X | X | 6 | 1 | R, M | ||
Mimidae | Melanotis caerulescensa | O | X | 2 | 0 | R | ||
Fringillidae | Euphonia elegantissima | F | X | 1 | 0 | R | ||
Parulidae | Setophaga dominica | I | X | 1 | 0 | M | ||
Basileuterus belli | I | X | 3 | 0 | R | |||
Myioborus pictus | I | X | 1 | 22 | R | |||
Cardinalidae | Piranga leucoptera | F, I | X | X | 0 | 3 | R | |
Habia fuscicauda | G, I, F | X | 1 | 0 | R | |||
Passerina ciris*** | G, I, F | X | 2 | 0 | T | |||
IS, immature seeds; MS, mature seeds; and LQ, low seed quality; Presence (X) and relative abundance (N) are reported. Bird nomenclature follows the American Ornithological Society (Chesser et al. 2019). Trophic guild: F, frugivore; G, granivore; I, insectivore; N, nectarivore; O, omnivore; C, carnivore. Residence status: R, breeding resident; M, winter migrant; T, transient Endemism: a endemic to Mexico and b restricted distribution Endangered species according to NOM-059 (SEMARNAT 2010) * Endangered; **Threatened; and *** Special Protection |
q=0 | Cl | q=1 | Cl | Sc | |
Study forest | |||||
El Gosco | 19 | 3.48 | 13.8 | 3.52 | 0.83 |
Medio Monte | 29 | 3.53 | 14.11 | 2.64 | 0.94 |
Phenological process | |||||
IS | 24 | 4.07 | 10.09 | 2.39 | 0.93 |
MS | 15 | 1.71 | 10.16 | 1.2 | 0.88 |
LQ | 11 | 2.36 | 10 | 2.33 | 0.82 |
El Gosco | |||||
IS | 10 | 2.81 | 7.67 | 2.7 | 0.8 |
MS | 7 | 1.93 | 6.3 | 1.7 | 0.88 |
LQ | 7 | 1.97 | 6.61 | 2.11 | 0.49 |
IS | 10 | 7.67 | 7.67 | 2.7 | 0.8 |
MS | 7 | 6.3 | 6.3 | 1.7 | 0.88 |
Medio Monte | |||||
IS | 17 | 2.81 | 7.89 | 1.68 | 0.96 |
MS | 13 | 3.8 | 8.99 | 2.73 | 0.86 |
LQ | 7 | 2.76 | 5.85 | 3.02 | 0.56 |
IS | 17 | 2.81 | 7.89 | 1.68 | 0.96 |
MS | 13 | 3.8 | 8.99 | 2.73 | 0.86 |
IS, immature seeds; MS, mature seeds; and LQ, low seed quality |