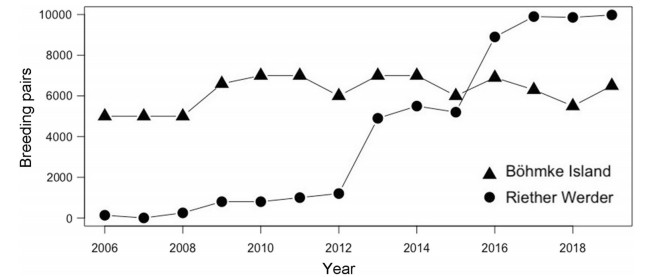
Citation: | Shi Li, Dan Li, Lishi Zhang, Weiping Shang, Bo Qin, Yunlei Jiang. 2021: High levels of genetic diversity and an absence of genetic structure among breeding populations of the endangered Rufous-backed Bunting in Inner Mongolia, China: implications for conservation. Avian Research, 12(1): 7. DOI: 10.1186/s40657-020-00236-3 |
The Rufous-backed Bunting, Emberiza jankowskii, is an endangered species that is primarily distributed in Inner Mongolia, China. The main threats to the continued persistence of this species are habitat loss and degradation. However, the impact of population loss on genetic diversity remains unclear. To support future conservation and management efforts, we assessed the genetic diversity and population structure of E. jankowskii using mitochondrial DNA and microsatellites.
Blood samples were collected from 7‒8-day-old nestlings in Inner Mongolia, China between May and August of 2012 and 2013. Mitochondrial DNA sequences and microsatellite markers were used to assess the genetic diversity, genetic structure and inbreeding of E. jankowskii. The results of genetic diversity and inbreeding were compared to other avian species.
We found an unexpectedly high level of genetic diversity in terms of mitochondrial DNA and microsatellite compared to other avian species. However, there were high levels of gene flow and minimal genetic structuring, among the fragmented breeding populations ofE. jankowskii in Inner Mongolia. These findings suggest that E. jankowskii in Inner Mongolia is a metapopulation. Despite the high genetic diversity of E. jankowskii, local populations in each small patch remain at risk of extinction due to habitat loss. In addition, the E. jankowskii population has a high risk of inbreeding.
To minimize further loss of genetic diversity of this endangered species, we suggest that the E. jankowskii in Inner Mongolia should be considered as a protected species for management purposes. Conservation efforts should concentrate on E. jankowskii habitat management. This may be most effectively achieved by protecting the current breeding habitats and prohibiting over-grazing.
Many animal species show philopatric behavior (Greenwood 1980), in which we distinguish between "natal" and "breeding" philopatry (Pearce 2007). "Natal philopatry" means not dispersing far from or returning to the own birthplace for reproduction (Pearce 2007), while "breeding philopatry" means that individuals return to the same area for reproduction every year (Shields 1982; Pearce 2007). Many colonially breeding seabirds such as Herring Gulls (Larus argentatus; Tinbergen 1961), Ring-billed Gulls (L. delawarensis; Southern 1977; Southern and Southern 1980), Sabine's Gulls (Xema sabini; Stenhouse and Robertson 2005), Black-legged Kittiwakes (Rissa tridactyla; Coulson and White 1958), and Common Terns (Sterna hirundo; Austin 1949) do not only show philopatry to a colony, but also to a special place within that colony, which is called site tenacity (Austin 1949; McNicholl 1975) or nest site tenacity (Fairweather and Coulson 1995) and can be understood as a very strong form of breeding philopatry. Strong breeding philopatry is also correlated with a high probability of breeding with the same partner for several years, whereas low breeding philopatry normally leads to mating with a new partner every year (Cézilly et al. 2000). Breeding philopatry is well known for Black-headed Gulls (Chroicocephalus ridibundus; Makatsch 1952), and is explained by a strong natal philopatry (Prévot-Julliard et al. 1998).
On the contrary, there are many reports of ringed birds that breed in colonies at large distances to their own hatching place. For example, recoveries of Black-headed Gulls breeding in Mecklenburg-Western Pomerania, although being ringed as nestlings in Belgium, the Netherlands, Great-Britain, Denmark, Poland, Lithuania, Estonia, or Sweden (Heinicke et al. 2016).
In this study, we investigated nest site tenacity and mate fidelity in the Black-headed Gull (C. ridibundus) in two different colonies at the German Baltic coast, which are very similar in their habitat conditions, but different in their stability. The colony on Riether Werder (53°42′22″ N, 14°15′38″ E) was newly established in 2006 (Heinicke et al. 2016), and has grown to the largest colony of Black-headed Gulls in Germany (Herrmann 2018). The colony on Böhmke Island (53°56′43″ N, 14°1′57″ E) exists since the 1950s (Nehls and Büttner 1973) and has had a stable number of breeding pairs during the last decade (numbers of breeding pairs in both colonies are shown in Fig. 1).
Long term ringing studies have shown that birds of both colonies show a strong breeding philopatry (Heinicke et al. 2016). However, the increase in breeding pairs on Riether Werder may lead to higher dynamics and instability in the colony as the growth affected the shape of the colony, its location, and the size of the occupied area on the island.
We therefore tested, if there are any differences in nest site tenacity and mate fidelity of Black-headed Gulls between these colonies. We expected that nest site tenacity is stronger in the stable colony at Böhmke Island than in the growing colony at Riether Werder, and due to the correlation between breeding philopatry and mate fidelity (Cézilly et al. 2000), we expected mate fidelity to be stronger in the stable colony at Böhmke Island as well. To investigate if the conditions of the nest location within a colony also play a role for nest site tenacity and mate fidelity, we also compared sites in the center with sites at the edge of each colony.
To ensure a comparable investigation of nest site tenacity, two study areas were chosen at the beginning of the breeding season 2016 in each colony. Each of these study areas had a size of 10 m × 10 m. They were located in the center (study area A) and at the edge (study area B) of a colony, respectively. As center we defined the area, where the first arriving Black-headed Gulls start to build their nests, as these are the areas with the highest nest density as well. Unexpectedly, the colony on Riether Werder grew on 3900 breeding pairs (+ 72%) compared to the previous year, so that our area B was no longer being located at the colony edge at the end of the breeding season 2016. Unfortunately, it was not possible to relocate area B after we had started our data collection. In 2017, area B was in fact in the center of the colony, showing an even higher nest density than study area A (see Table 1).
Study area | Nests 2016 | Nests 2017 | Change between years (%) |
Riether Werder A | 53 | 68 | +28.3 |
Riether Werder B | 53 | 84 | +58.5 |
Riether Werder A+B | 106 | 152 | +43.4 |
Böhmke Island A | 49 | 55 | +12.2 |
Böhmke Island B | 29 | 18 | −37.9 |
Böhmke Island A+B | 78 | 73 | −6.4 |
Total | 184 | 225 | +22.3 |
In 2017, due to weather and wind conditions, both colonies faced much higher water levels than in 2016. On Böhmke Island, the lower parts of the island, including parts of study area B, were flooded at the beginning of the breeding season. On Riether Werder, flooding was not an issue, because the colony is protected by a dike. However, due to heavy rainfall, large puddles emerged behind the dike contributing to the shift of the colony and re-definition of study area B from the edge to the center of the colony.
All nests in our study areas were marked with numbered wooden poles, which were stuck in the ground about 20 cm from each nest. Breeding birds inside the study areas were caught with walk in cage traps during the incubation period (May and early June). To prevent cooling of the eggs during catching, trapping attempts were limited to a maximum of 30 min per nest. All caught birds were marked with rings of the Hiddensee bird ringing center, and their sex was determined using its body measurements. According to different ringing protocols used in the long-term projects at the two islands, the formula by Holz and Starke (1984) was used for sex determination at Böhmke Island, while the head-bill-length after Baker (1993) was used at Riether Werder.
Due to the individual marking of nests and birds we were able to document which bird bred with which partner in which nest. In 2017, we repeated the whole procedure, and were able to determine if birds were breeding again in our study area, and if they still had the same partner.
The number of nests and caught individuals increased in all study areas except of study area B (edge) on Böhmke Island, where less pairs were recorded in the second study year (Tables 1, 2).
Study area | Captured individuals 2016 | Captured individuals 2017 | Recaptured individuals (m/f) | Recapture rate (%) |
Riether Werder A | 72 | 99 | 14 (8/6) | 19.4 |
Riether Werder B | 82 | 131 | 22 (9/13) | 26.8 |
Riether Werder A+B | 154 | 230 | 36 (17/19) | 23.4 |
Böhmke Island A | 82 | 100 | 47 (22/25) | 57.3 |
Böhmke Island B | 45 | 30 | 13 (8/5) | 28.9 |
Böhmke Island A+B | 127 | 130 | 60 (30/30) | 47.2 |
Total | 281 | 360 | 96 (47/49) | 34.2 |
A direct estimation of general nest site tenacity is not easy due to the difficulties tracing dispersing birds, especially if they disperse to other colonies. Considering only ringing data from our study sites, we were not able to establish if non-recaptured birds have died, or not showed nest site tenacity. Assuming however, that mortality rates were similar for birds at all study areas, recapture rates at each study area can be used as indirect measurement of nest site tenacity. As expected, the recapture rate was significantly higher in the stable colony on Böhmke Island (47.2%) than in the newly established and fast-growing colony on Riether Werder (23.4%), Pearson's χ2-test: χ2 (1, N = 281) = 17.628, p < 0.001. On Böhmke Island, the recapture rate was significantly higher in study area A representing the colony center (57.3%), than in study area B (28.9%), Pearson's χ2-test: χ2 (1, N = 127) = 9.421, p = 0.002.
These results indicate that Black-headed Gulls in our colonies showed a tenacity to special places within the colony. Similar behavior was described for other colonially breeding seabirds (S. hirundo, Austin 1949; R. tridactyla, Coulson and White 1958; L. argentatus, Tinbergen 1961; L. delawarensis, Southern 1977; Southern and Southern 1980).
We assume, that the annual shift in size and shape of the colony on Riether Werder was the main reason for the lower recapture rates in our study areas on this island. These changes were mainly caused by the annual growth of the colony over the past few years. Additionally, due to strong rain in April and early May in 2017, large puddles covered parts of the colony, inducing more changes in it. These shifts were also the main reason, why study area B on Riether Werder does not longer represented the edge of the colony. Consequently, the recapture rates were more similar between the study areas on Riether Werder than at Böhmke Island. The puddles, covering a large proportion of previously occupied areas, also led to a higher nest density in other parts of the colony. In both study areas we found a strong increase in the number of nests (see Table 1). These changes occurring in the colony on Riether Werder represented a disturbance for our experimental setup on the one hand, but, on the other hand, were also a confirmation of the higher dynamics of this colony.
Some other factors may have contributed to the lower degree of nest site tenacity found in the colony on Riether Werder as well. We speculate that the fast-growing colony contained a much higher proportion of younger birds including first-time breeders than the colony on Böhmke Island. Younger birds normally have lower reproductive success than older birds, which are more experienced in breeding and raising offspring (Lack 1968). For other philopatric bird species, it has been indicated that birds with no or low breeding success show a higher tendency to change their breeding place than successful ones (R. tridactyla, Naves et al. 2006), and that nest site tenacity increases with age and experience of the birds (S. hirundo, Austin 1949). We think, that both, the annual change in the colony, and the age and experience of the breeding birds contributed to the differences in the degree of nest site tenacity found in the two colonies.
As already stated, a differentiation of our study areas between center and edge of the colony was not possible on Riether Werder. However, we could observe significant differences on Böhmke Island (recapture rate center: 57.3%; recapture rate edge: 28.9%; Pearson's χ2-test: χ2 (1, N = 127) = 9.421, p = 0.002). These differences may have been a consequence of high water levels causing partial flooding of the colony margins at the beginning of the breeding season 2017. Therefore, a lower number of nests were found in study area B in the second study year, whereas the nest density in the center was slightly higher than in the year before (see Table 1). The flooding occurred early in the breeding season, and although we did not find flooded nests and the area was dry during the main breeding season, just a few birds nested there, possibly reflecting avoidance of the risk of a second flood. Due to this risk, the lower parts of the island, hence the edges of the colony could be declared as low-quality nest sites. In a study by Dexheimer and Southern (1974), flooding of nests was the main reason for lower breeding success and survival rates of Ring-billed Gull chicks at the edge of a colony, compared to its center.
Predation also represents a higher risk to nests located at the colony edge (Tenaza 1971). Although our study islands do not have terrestrial predators, we documented predation of adult birds by raptors such as Peregrine Falcon (Falco peregrinus) and Goshawk (Accipiter genitilis), of chicks by White-tailed Eagle (Haliaeetus albicilla), Marsh Harrier (Circus aeruginosus), and Kites (Milvus migrans and Milvus milvus), and of eggs by Ravens (Corvus corax) and Crows (Corvus corone cornix). We observed that all these raptors tended to hunt at the edges of the colony, because the communal defense behavior of the breeding birds was much stronger in the center. Therefore, we assume that even in our colonies, predation risk is higher at the edges.
Even without flooding or predation, breeding success and survival rates of chicks have been found to be lower at colony edges than in the center (Patterson 1965; Coulson 1968; Dexheimer and Southern 1974), and were explained by the fact, that younger, less experienced, and weaker individuals tend to breed at the low-quality nest sites at the edges of a colony, whereas the high-quality nest sites in the center of a colony are occupied by the strongest and more experienced birds. We assume the same situation happening in our colonies, because each year, the birds arriving first settle in the central parts, and older Black-headed Gulls arrive earlier at the breeding place than younger birds (Makatsch 1952). So here again, the age and experience of the birds may influence nest site tenacity.
The more than twice higher recapture rate of study area A on Böhmke Island compared to the other three study areas (Table 2) is remarkable. Both central areas on Riether Werder (A and B), showed recapture rates slightly lower than the recapture rate at the colony edge on Böhmke Island (B), reflecting a degree of nest site tenacity in the center of the colony on Riether Werder similar to the one at the edge on Böhmke Island. We believe, that due to its annual changes, the colony on Riether Werder has not established a real center, yet. The colony has areas with higher and lower nest density, but as drone pictures showed (Grenzdörffer 2013, 2014, 2015, unpublished), these areas are changing between seasons. The inexistence of an established center would explain the lower nest site tenacity found on Riether Werder.
The general advantages and disadvantages of philopatry have often been discussed (Greenwood 1980), but we want to highlight some factors that may play a major role for the species under study. According to McNicholl (1975), breeding philopatry reduces the susceptibility to predators and other destructive factors due to the familiarity of birds to dangers of the habitat. At both islands, due to predator control, terrestrial predators do not occur, implying that the birds only need to practice communal defense against avian predators year after year. Another threat, especially on Böhmke Island, is represented by high water levels. The colony on Böhmke Island is not protected by a dike, and therefore, nests located in low areas are especially exposed to flooding. If the birds are familiar with this risk, they are able to choose safer nest sites in the higher parts of the island. A second important advantage of breeding philopatry is, that if both partners return to the previous breeding area, they can again mate with each other, which may lead to a higher breeding success (Coulson 1966, see mate fidelity).
We did not find indications of sex-based differences in the nest site tenacity of Black-headed Gulls. Males and females have been recaptured at similar numbers (Table 3). However, it might be possible that there is a sexual difference in the natal philopatry of Black-headed Gulls as it was shown for Herring Gulls (Chabrzyk and Coulson 1976), Red-billed Gulls (Chroicocephalus novaehollandiae scopulinus; Mills 1973), and Black-legged Kittiwakes (Wooller and Coulson 1977). Sex based differences in natal philopatry may be important for inbreeding avoidance (Greenwood 1980) and might be an explanation for the strong degree of natal philopatry in the Black-headed Gull found by Prévot-Julliard et al. (1998) on the one hand and the dispersal over large distances described by Heinicke et al. (2016) on the other hand.
Study area | Group 1 | Group 2 | Group 3 | Group 4 | Fidelity (%) | Divorce rate (%) |
Riether Werder A | 4 | 0 | 0 | 10 | 100.0 | 0 |
Riether Werder B | 8 | 1 | 0 | 13 | 88.9 | 0 |
Riether Werder A+B | 12 | 1 | 0 | 23 | 92.3 | 0 |
Böhmke Island A | 28 | 5 | 2 | 12 | 80.0 | 5.7 |
Böhmke Island B | 10 | 1 | 0 | 2 | 90.9 | 0 |
Böhmke Island A+B | 38 | 6 | 2 | 14 | 82.6 | 4.3 |
Total | 50 | 7 | 2 | 37 | 84.7 | 3.8 |
Groups 1: same partner in both years; Group 2: new partner in the second year (previous partner untraceable); Group 3: new partner in the second year (previous partner present); Group 4: no data available because partner was not caught in both years |
We documented an interesting case of a pair with strong nest site tenacity. These individuals bred successfully on Böhmke Island (study area A) in 2016, but lost their clutch in 2017 to predation. Two weeks later, both birds were caught on a second nest just behind the first one. Although it has been shown that birds not breeding successfully tend to choose a new breeding place (Greig-Smith 1982; Naves et al. 2006), this pair built its new nest at the same site that failed. This case might be explained by a possible long-standing breeding success at this nest site. Similar cases have been documented in Common Terns by González-Solís et al. (1999).
To analyze mate fidelity, we sorted all recaptured individuals into four groups: (1) bred with same partner in both years; (2) bred with new partner in the second year, previous partner untraceable; (3) bred with new partner in the second year, previous partner present; (4) no data available because partner was not caught in both years.
Individuals of group 4 were not considered for the analysis. We calculated the proportion of birds showing mate fidelity as a percentage of individuals of group 1 relative to all individuals of groups 1, 2 and 3. Additionally, we calculated a "real divorce rate" where we neglected the birds of group 2 by calculating the percentage of individuals of group 3 relative to all individuals of groups 1 and 3.
For an adequate interpretation of our results on mate fidelity we have to stress the limitation of our method considering only individuals also showing nest site tenacity. We do not have mate fidelity information for individuals which changed their breeding places. However, it is unlikely that such individuals will show the same degree of mate fidelity as birds which returned to a previously used breeding place. According to Cézilly et al. (2000), birds changing their breeding places have a much lower probability to breed again with the same partner than birds showing nest site tenacity. However, in some species pairs were documented which reunited after a breeding area change (Stenhouse and Robertson 2005).
After recording the highest nest site tenacity levels at the colony center on Böhmke Island, it was no surprise to find the highest number of birds showing mate fidelity there as well. It seems however, that even if less birds showed nest site tenacity at other study areas, a large proportion of these did show mate fidelity as well. In total, from 34 pairs of which we caught both partners in both years, we documented 50 individuals (25 pairs) that reunited with their previous partner, and only 9 birds breeding with a new partner. This is a proportion of 84.7% of birds showing mate fidelity. A similar proportion of ≥ 80% of birds showing mate fidelity was found in all four study areas (Table 3), the difference between the study areas was not significant (p = 0.936, two-sides Fisher's Exact Test).
Of the nine birds which bred with a new partner, we were not able to detect the previous partner in the colonies for seven of them. Unfortunately, we do not know if they died or dispersed to another colony. The absence of their previous partner may have prompted returning individuals to choose a new one, therefore they were excluded for the estimations of divorce rates. We were able to document only one real "divorce", hence two birds mating with new partners, although their previous ones were still present. These two birds bred together in 2016 in study area A on Böhmke Island, and each of them bred in the same study area in 2017 with a new partner. These new partners have not been documented in the study area in the previous year. Comparing this case to the 50 individuals showing mate fidelity resulted in a "divorce rate" of 3.8%.
Mate fidelity may ensure earlier breeding start due to a reduced search time for new breeding places and mating partners. Moreover, studies have shown that birds breeding together several times have a significantly higher breeding success as pairs breeding together for the first time (Coulson 1966). Hamer et al. (2002) explain this by a higher efficiency for birds that have bred together before, as these individuals are able to cooperate and to coordinate breeding and raising of the offspring better than individuals not having this experience together.
We also observed two interesting mate choice cases. One consisting of two females and one male which bred on a nest with six eggs in study area A on Riether Werder in 2016, and another pair consisting of two females breeding in both years in study area A on Böhmke Island. Of course, mistakes in the sexing method could not be excluded, but at least the birds of the female-female pair on Böhmke Island were caught in both years, and both birds have been morphologically identified as females twice. Female-female-male trios as well as female-female pairs have been found in other species, such as the Black-legged Kittiwake (Coulson and Thomas 1985). Fitch and Shugart (1983) estimated a proportion of female-female-male trios in a Herring Gull colony of 0.6–1.0% and found no significant difference between the mate fidelity of female–female–male trios and normal pairs. Unfortunately, none of the birds from the trio on Riether Werder was detected there in the following year. The female-female pair on Böhmke Island, however, showed nest site tenacity as well as mate fidelity, which Kovacs and Ryder (1981) also documented for female–female pairs of Ring-billed Gulls. The female–female pair on Böhmke Island bred successfully in both years. According to Hamer et al. (2002) in female–female pairs the eggs are fertilized by extra-pair copulations with males which did not contribute to breeding and raising of the offspring. Both phenomena, female-female-male trios as well as female-female pairs seem to be a result of an uneven sex-ratio found in gull and tern populations (Hamer et al. 2002).
First of all, we want to thank Frank Joisten and Wilfried Starke, caretakers of Riether Werder and Böhmke Island, for all their help in implementing this study in their long-term ringing studies and providing all necessary data and information about the islands and the colonies. We also want to thank Dr. Görres Grenzdörffer for providing the unpublished original Data of the dronebased count from Riether Werder in 2017. Last but not least we want to thank all assistants and volunteer ringers who enabled the study to be done at the same time on the two islands.
The study was designed by SP and ASO. Data was collected in the long-term projects by volunteer ringers (including the authors), and analyzed and interpreted by SP and ASO. The first version of the manuscript was written by SP and edited for publication by SP and ASO. Both authors read and approved the final manuscript.
All data generated or analyzed during this study are included in this published letter, individual ringing data is available from the corresponding author on request.
The study was implemented in authorized long-term ringing projects of wild birds on both islands, and performed by licensed ringers of the Hiddensee Bird Ringing Center.
Not applicable.
The authors declare that they have no competing interests.
Du Y. Genetic diversity of Varied Tit (Parus varius) inferred with mitochondrial DNA control region and nuclear microsatellite markers. Master's Thesis. Shenyang, China: Liaoning University; 2011.
|
Frankel OH, Soulé ME. Conservation and evolution. Cambridge: Cambridge University Press; 1981.
|
Freeland JR, Kirk H, Petersen S. Molecular ecology. 2nd ed. Chichester: Wiley; 2011.
|
Goudet J. FSTAT, version 2.9.3, a program to estimate and test gene diversities and fixation indices. Lausanne: Lausanne University; 2001.
|
Graur D, Li WH. Fundamentals of molecular evolution. 2nd ed. Sunderland: Sinauer Associates; 2000.
|
Hamrick JL, Godt MJW, Murawshi DA, Loveless MD. Correlations between species traits and allozyme diversity: implications for conservation biology. In: Falk DA, Holsinger KE, editors. Genetics and conservation of rare plants. New York: Oxford University Press; 1991. p. 75-86.
|
He LP. Isolation of polymorphic microsatellite loci and analysis of genetic diversity in Crested Ibis (Nipponia nippon). Ph. D Thesis. Hangzhou: Zhejiang University; 2007.
|
Jamieson IG, Wallis GP, Briskie JV. Inbreeding and endangered species management: is New Zealand out of step with the rest of the world? Conserv Biol. 2006;20: 38-47.
|
Lande R. Extinction risks from anthropogenic, ecological, and genetic factors. In: Landweber LF, Dobson AP, editors. Genetics and the extinction of species: DNA and the conservation of biodiversity. Princeton: Princeton University Press; 1999. p. 1-22.
|
Matocq MD, Villablanca FX. Low genetic diversity in an endangered species: recent or historic pattern? Biol Conserv. 2001;98: 61-8.
|
Rousset F. Genetic structure and selection in subdivided populations (MPB-40). Princeton: Princeton University Press; 2004.
|
Schonewald-Cox CM, Chambers SM, MacBryde B, Thomas WL. Genetics and conservation: a reference for managing wild animals and plant populations. Menlo Park: Benjamin/Cummings Publishings; 1983.
|
Slatkin M. Gene flow and population structure. In: Real LA, editor. Ecological genetics. Princeton: Princeton University Press; 1994. p. 3-17.
|
Swofford DL. Paup: Phylogenetic analysis using parsimony (and other methods), 4.0b. Sunderland: Sinauer Associates; 2001.
|
Wonke G, Wallschläger D, Moll K, Tiedemann R. Ten new microsatellite loci for the yellowhammer (Emberiza citrinella) and their cross-species applicability among related taxa. Mol Ecol Resour. 2007;7: 1278-80.
|
Zhang WW. Genetic diversity and classification research of Japanese Marsh Warbler (Megulus palustris sinensis). Master's Thesis. Harbin: Northeast Forestry University; 2007.
|
Zhao XQ. Population structure of Japanese Marsh Warbler (Megalurus pryeri) by microsatellite. Master's Thesis. Harbin: Northeast Forestry University; 2012.
|
1. | Fang Wang, Xingzhong Yuan, Yingjun Sun, et al. Species distribution modeling based on MaxEnt to inform biodiversity conservation in the Central Urban Area of Chongqing Municipality. Ecological Indicators, 2024, 158: 111491. DOI:10.1016/j.ecolind.2023.111491 |
2. | Isaac Morales-Yañez, Ricardo Rodríguez-Estrella, Ana Bertha Gatica-Colima. Selección de sitios de anidación por el águila real Aquila chrysaetos (Linnaeus, 1758) (Accipitriformes: Accipitridae) en Janos, Chihuahua, México. ACTA ZOOLÓGICA MEXICANA (N.S.), 2023. DOI:10.21829/azm.2023.3912564 |
3. | Alessandro Berlusconi, Alessio Martinoli, Lucas A. Wauters, et al. Year-round multi-scale habitat selection by Crested Tit (Lophophanes cristatus) in lowland mixed forests (northern Italy). Avian Research, 2022, 13: 100058. DOI:10.1016/j.avrs.2022.100058 |
4. | Dimitar Demerdzhiev. Breeding parameters and factors influencing the reproduction of an expanding Long-legged Buzzard (Buteo rufinus) population under high breeding density conditions. Journal of Ornithology, 2022, 163(2): 405. DOI:10.1007/s10336-022-01967-4 |
5. | Maria Solanou, Eirini Trypidaki, Elisavet Georgopoulou, et al. Selection of Nesting Habitat and Insular Niche Separation of Two Sympatric Aquila Species. Diversity, 2022, 14(12): 1136. DOI:10.3390/d14121136 |
Study area | Nests 2016 | Nests 2017 | Change between years (%) |
Riether Werder A | 53 | 68 | +28.3 |
Riether Werder B | 53 | 84 | +58.5 |
Riether Werder A+B | 106 | 152 | +43.4 |
Böhmke Island A | 49 | 55 | +12.2 |
Böhmke Island B | 29 | 18 | −37.9 |
Böhmke Island A+B | 78 | 73 | −6.4 |
Total | 184 | 225 | +22.3 |
Study area | Captured individuals 2016 | Captured individuals 2017 | Recaptured individuals (m/f) | Recapture rate (%) |
Riether Werder A | 72 | 99 | 14 (8/6) | 19.4 |
Riether Werder B | 82 | 131 | 22 (9/13) | 26.8 |
Riether Werder A+B | 154 | 230 | 36 (17/19) | 23.4 |
Böhmke Island A | 82 | 100 | 47 (22/25) | 57.3 |
Böhmke Island B | 45 | 30 | 13 (8/5) | 28.9 |
Böhmke Island A+B | 127 | 130 | 60 (30/30) | 47.2 |
Total | 281 | 360 | 96 (47/49) | 34.2 |
Study area | Group 1 | Group 2 | Group 3 | Group 4 | Fidelity (%) | Divorce rate (%) |
Riether Werder A | 4 | 0 | 0 | 10 | 100.0 | 0 |
Riether Werder B | 8 | 1 | 0 | 13 | 88.9 | 0 |
Riether Werder A+B | 12 | 1 | 0 | 23 | 92.3 | 0 |
Böhmke Island A | 28 | 5 | 2 | 12 | 80.0 | 5.7 |
Böhmke Island B | 10 | 1 | 0 | 2 | 90.9 | 0 |
Böhmke Island A+B | 38 | 6 | 2 | 14 | 82.6 | 4.3 |
Total | 50 | 7 | 2 | 37 | 84.7 | 3.8 |
Groups 1: same partner in both years; Group 2: new partner in the second year (previous partner untraceable); Group 3: new partner in the second year (previous partner present); Group 4: no data available because partner was not caught in both years |