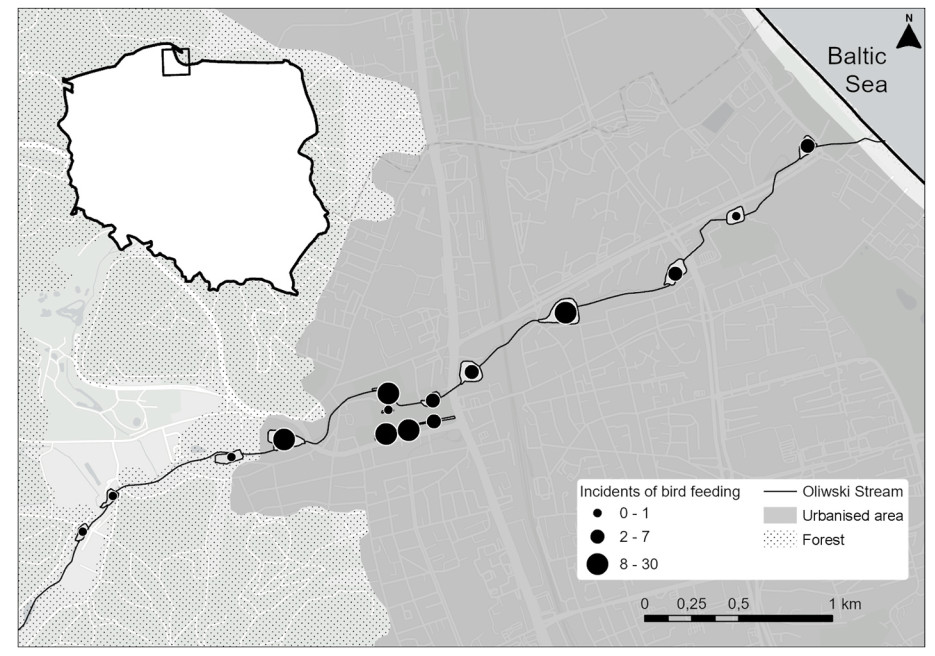
Citation: | Marta Witkowska, Wojciech Wesołowski, Martyna Markiewicz, Jonasz Pakizer, Julia Neumann, Agnieszka Ożarowska, Włodzimierz Meissner. 2024: The intensity of supplementary feeding in an urban environment impacts overwintering Mallards (Anas platyrhynchos) as wintering conditions get harsher. Avian Research, 15(1): 100205. DOI: 10.1016/j.avrs.2024.100205 |
Although urbanization poses various threats to avifauna, some bird species, including Mallards (Anas platyrhynchos), choose towns and cities as their wintering habitats, possibly due to favourable temperatures and abundant anthropogenic food. In this study, we investigated how population dynamics changed in relation to winter harshness and intensity of supplementary bird feeding in Mallard, a dabbing duck species well adapted to an urban environment. We surveyed 15 city ponds for five consecutive years, counting overwintering individuals and incidents of bird feeding conducted by the citizens of Gdańsk. Number of Mallards observed in the studied area fluctuated both annually and within seasons, with on average 327 (± SD = 108.5) individuals and a male-biased sex ratio reported. We observed a gradient of feeding intensity on the surveyed ponds, with number of feeding incidents ranging from 0 to 30 in a day. The results indicated that the number of Mallards increased with the bird feeding intensity, independently of the size of the studied ponds, and both males and females were similarly attracted by food provisioning. More severe wintering conditions, expressed by the percentage of the ice cover of studied ponds resulted in more birds wintering in the urban area. This effect was more pronounced in females compared to males, possibly due to females having a lower ability to withstand severe wintering conditions, forcing them to relocate towards urban areas. Our results confirm that urbanized areas may serve as wintering grounds for birds, due to additional food resources available there and milder wintering conditions compared to surrounding rural areas.
The ongoing urbanization of the world, with the growing number and areas of cities, as well as the size of the human population living in them, rapidly influences the natural environment and populations of animals. Usually, the impact of urbanisation on wildlife is reported as negative, since it results in the degradation or loss of habitats and extinction of species, leading to a decline in biodiversity (Clergeau et al., 2006; Aronson et al., 2014; Wang et al., 2020; Zhu et al., 2020). For instance, birds within urbanized areas are at risk of mortality due to collisions with built structures (Doren et al., 2021), the presence of domesticated predators (Loss and Marra, 2017; Pavisse et al., 2019), and increased transmission of potentially pathogenic microorganisms (Meissner et al., 2015a). Other negative effects reported point out to the limited birds’ vocal communication, due to higher level of noise compared to rural areas (Brumm, 2004; Slabbekoorn and Ripmeester, 2008), as well as shifts in their daily and annual cycle events caused by the artificial light pollution present in the cities (Dominoni, 2015). At the same time, cities provide a new type of environment that may be utilized by some species either by colonizing the urban spaces and constructions or using green spaces inside cities that are intended to welcome wildlife and allow citizens to interact with it (Chace and Walsh, 2006; González-Lagos et al., 2021). Such interaction was reported to be beneficial for people living in the cities, with supplementary feeding of animals being one of the most commonly practiced activities of this kind, bringing citizens into closer contact with wildlife (Cox and Gaston, 2016). As food resource availability is a key factor determining animal population structure and distribution, the provision of anthropogenic food both intentional (e.g., feeders in private gardens, feeding wildlife in parks and public gardens) and unintentional (e.g., trash with leftover food, remains from feeding domesticated animals) may locally improve feeding conditions and therefore increase the population size and density (Fuller et al., 2008; Cox and Gaston, 2018; Plummer et al., 2019).
The Mallard (Anas platyrhynchos) is a dabbling duck species that has adapted to the urban environment, and extensively uses the waterbodies located there during different stages of its annual cycle (Meissner et al., 2015b; Jarman et al., 2020; Kopij, 2020). For overwintering individuals, cities generally provide higher ambient temperatures (Oke, 1982; Kalnay and Cai, 2003), keeping the surfaces of the waterbodies from freezing, and therefore allowing Mallards to obtain their natural food. This, together with the supplementary feeding provided by citizens, may add up to abundant food resources offered by the urban environment. Additionally, higher ambient temperatures within cities may create fewer constraints on keeping the metabolic rate essential for maintaining the stable body temperature of endothermic birds. This in turn lowers the costs of maintenance of individuals overwintering in the cities, compared to their conspecifics wintering in more natural habitats (Tryjanowski et al., 2015). The ambient temperature changes and winter harshness were reported as the main factors influencing the demographic parameters of the Mallard population, such as its size (Meissner et al., 2015b; Schummer et al., 2010), sex-ratio (Pattenden and Boag, 1989; Meissner and Witkowska, 2023), and survival (Bergan and Smith, 1993; Manikowska-Ślepowrońska and Meissner, 2022).
In urbanized areas, Mallards gather in large numbers in sites where people feed them (Engler et al., 1988; Avilova and Eremkin, 2001). Yet, the studies of urban overwintering waterbirds usually only speculate about the importance of supplementary feeding and its consequences for the population, without including it as a factor in the research design (Avilova and Eremkin, 2001; Meissner and Witkowska, 2023; but see Polakowski et al., 2010). As the availability of food resources during winter is one of the key factors determining the distribution of birds (Crampton et al., 2011; Ciach and Fröhlich, 2017), including its effect in population studies would allow for obtaining more precise results. Indeed, consequences of the anthropogenic food provisioning during winter on birds’ populations were previously described, with the increase in population density as one of the effects reported (e.g., Fuller et al., 2008). Moreover, supplementary feeding may contribute to species range expansion (Greig et al., 2017) and increased diversity of bird communities (Plummer et al., 2019). However, those effects were reported for land birds using garden feeders and in particular passerines. In this study, we aimed to investigate the changes in the abundance of Mallards wintering in urban areas in relation to the winter harshness, described as a decrease in the ambient temperature or freezing of the ponds occupied by this species during winter. Moreover, we considered the effect of the intensity of supplementary bird feeding conducted on the local population of overwintering Mallards. We hypothesized that the number of individuals wintering in the urbanized area increases locally with harsher wintering conditions and increased food provisioning conducted by citizens.
The survey has been conducted on 15 different ponds located on the Oliwski Stream in Gdańsk (Poland 54°21′ N, 18°40′ E; Fig. 1, Appendix A Fig. S1) for five consecutive winter seasons since the winter of 2017/2018. We counted female and male Mallards separately, every two weekends, starting from the third weekend of December, until the second weekend of March, which resulted in seven counts each season. This protocol was not followed during the first, pilot season of winter 2017/2018, where we counted birds on five occasions only, from the last weekend of January to the second weekend of March (Appendix A Table S1). Nonetheless, we included the data collected during that season in our analysis, as it covered the majority of the established studied period. Each counting event started at 8:00 a.m. in the same place, being the central pond in the middle of Oliwski Stream, followed by one person conducting counts on seven ponds located upstream and the other person surveying eight ponds located downstream from the starting point. The total time of the census did not exceed 3 h. Over the studied period, each pond was surveyed 33 times, providing 990 data entries (495 entries for each sex).
To establish the intensity of anthropogenic bird feeding conducted on each pond (later referred to as ‘IAF’) we observed citizens feeding Mallards and other waterbirds (i.e., gulls, Mute Swans Cygnus olor, and other species of ducks) occupying a given pond during the weekend (Appendix A Fig. S1). During those observations, we counted the incidents of birds feeding for each pond separately. We were unable to assess the amount of food provided and ensure that only Mallards, among other bird species present, were being fed. Therefore, our observations provided only a general indicator of IAF. The surveys were conducted during the last season of the study in the winter 2021/2022, on the same days as counts of wintering Mallards. We did not conduct surveys of feeding during bad weather conditions discouraging people from spending their time outside. We considered 9:00 a.m. until 3:00 p.m. to be rush hours for citizens' outdoor activities, and within that period, we established eight, 45-min-long intervals, in which we conducted our observations. In each interval, a given pond was observed for 30 min and the rest of the remaining time was used by the observer to commute from one pond to another. The sequence of ponds surveyed by an observer in consecutive time intervals was established at random, ensuring the collection of representative data and minimizing any time-related bias. On average all eight intervals of observations on a given pond were completed over three different days. Later we summed the number of incidents of birds feeding from eight intervals of observations and used it as an indicator of IAF for each one of the ponds. The used indicator was applied to data gathered before the winter of 2021/22, under the assumption that citizens had been using the studied area similarly over the studied years.
Along with the census of Mallards, we estimated the percentage of ice cover on the surface of surveyed ponds. Ambient temperature was calculated as a mean temperature from five days preceding the date of the survey, as the response of Mallards to a drop in temperature does not have to be immediate, and short-term chilling might not promote their movements (Sauter et al., 2010). The data on ambient temperature was taken from the reports of the meteorological station located at Gdańsk Rębiechowo Airport, located approximately 7 km from the studied site. The winters in the studied area were rather mild, as the mean winter temperature from five years of the study was 1.5 ℃, and in the case of only eight counting events the mean ambient temperature during the day was below 0 ℃ (Fig. 2, Appendix A Table S1).
To check how the population size of Mallards was influenced by winter harshness (ambient temperature or percentage ice cover) and IAF, we used the zero-inflated Generalized Linear Mixed Model with Negative Binomial distribution (where the variance increases quadratically with the mean; Hardin and Hilbe, 2018) and log-link function, computed with glmmTMB package in R (Brooks et al., 2017). First, we developed a global model including all considered parameters. The conditional part of this model as fixed parameters included IAF and either temperature or percentage of ice cover (due to strong correlation between those two parameters: Pearson correlation r = 0.85, p < 0.001), as well as sex of counted individuals, and interaction of sex with both IAF and temperature or percentage of ice cover. Pond, year, and sequence number of consecutive counting corresponding to the same weekend of a given month in different seasons were established as random parameters to control for their randomized effects. The zero-inflation model accounted for an equal probability of observing an excess zero for all observations. The global model's quality was evaluated using package performance in R (Lüdecke et al., 2021). We detected no over- or underdispersion in either global model (dispersion ratio = 0.475 and 0.468 for the global model including temperature and percentage of ice cover respectively). Both global models satisfactorily accounted for the excess of zeros in the data, where the ratio of predicted zeros to observed zeros was 0.87 in the model including temperature, and 0.93 in the model including the percentage of ice cover. The variance inflation factor (VIF) calculated for the fixed effects in conditional parts of both global models was less than five in all cases, indicating a lack of multicollinearity. Evaluating the structure of random effects included in global models revealed no issues with singularity and convergence.
In the set of candidate models predicting the number of individuals, we included two global models (with either temperature or percentage of ice cover), the null model including only intercept and no fixed parameters in the conditional part of the model, and reduced models established with backward elimination of insignificant variables from the global models. This process resulted in obtaining two reduced models. In the case of the global model with temperature as a fixed effect, we subsequently excluded the interaction of sex with IAF, the size of the pond, and the interaction of sex with temperature as spurious fixed effects. From the global model with the percentage of ice cover as a fixed effect excluded variables were the interaction of sex with IAF and the size of the pond. We used the Akaike Information Criterion for small sample size (AICc) and Akaike weights to rank the models in the set of candidate models, considering the model with the lowest AICc, the ΔAICc < 2, and the highest weights to be the most informative (Burnham and Anderson, 2004). Inference was then made from the best-fitted model. All statistical analysis was conducted in R 4.2.2 (R Core Team, 2021).
Each year we recorded on average 327 (± SD = 108.5) individuals; with 198 males (± SD = 63.2) and 129 females (± SD = 46.4) present in the studied area. The number of individuals varied both annually and within a season, with the average lowest and highest numbers of individuals detected in winter 2019/20 and 2020/21 respectively (Fig. 2B, Appendix A Table S2). The average number of bird feeding incidents observed was 9.4 (± SD = 9.76) for all 15 ponds. In the case of one pond, we observed a maximum number of 30 feeding incidents, and on 3 different ponds, we did not detect any bird feeding (Fig. 1).
The reduced model including percentage of ice cover was the most informative in the set of candidate models (Table 1). According to this model, the number of wintering Mallards could be explained by the percentage of ice cover, the IAF, and sex of individuals as well as interaction between sex and percentage of ice cover, while controlling for random effects of season, date of the census, and pond (Table 2). Although the inclusion of random effects increased the fit of models, the percentage of variance explained by them was low (Table 2). Generally, models including the percentage of ice cover instead of ambient temperature had higher support, although all models had an improved fit compared to the null model (Table 1).
Conditional model | R2 cond. | R2 marg. | AiCc | ΔAICc | wi | df. resid | Dev | |
Reduced (with ice) | N = ice + IAF + sex + ice * sex + r (year) + r (day) + r (pond) | 0.54 | 0.27 | 5514.7 | 0 | 0.669 | 894 | 5495.7 |
Global (with ice) | N = ice + IAF + sex + size + ice ∗ sex + IAF ∗ sex + r (year) + r (day) + r (pond) | 0.53 | 0.28 | 5517.3 | 2.6 | 0.331 | 892 | 5493.0 |
Reduced (with temp | N = temp + IAF + sex + r (year) + r (day) + r (pond) | 0.52 | 0.25 | 5701.9 | 187.2 | <0.001 | 927 | 5683.8 |
Global (with temp) | N = temp + IAF + sex + size + temp ∗ sex + IAF ∗ sex + r (year) + r (day) + r (pond) | 0.52 | 0.28 | 5702.6 | 187.9 | <0.001 | 924 | 5678.3 |
Null | N = r (year) + r (day) + r (pond) | 0.50 | 0 | 5798.2 | 283.5 | <0.001 | 930 | 5786.2 |
Model factors included: ice – ice cover on a given pond (%); temp – ambient temperature (℃); IAF – the intensity of anthropogenic birds feeding conducted on a given pond; sex – sex of counted individuals; size – size of given pond (m2); year - season, day – consecutive counting corresponding to the same weekend of a given month in different seasons; pond – given pond; r () – random factor; * – interaction between independent variables; Provided metrics: R2 cond. – conditional R2, R2 marg. – marginal R2; AICc – Akaike’s Information Criterion for small sample size; ΔAICc – the difference between AICc of a given model and the model with the lowest AICc; df. resid – residual degrees of freedom; Dev – deviance. |
Fixed effects | Variance explained (%) | Estimate | SE | z-value | p-value |
Zero-inflation model | |||||
Intercept | −3.214 | 0.232 | −13.86 | <0.001 | |
Conditional model | |||||
Intercept | 1.129 | 0.268 | 4.208 | <0.001 | |
IAF | 22.5 | 0.068 | 0.018 | 3.660 | <0.001 |
Ice | 1.0 | 0.002 | 0.001 | 2.658 | 0.008 |
sex (male) | 1.8 | 0.468 | 0.055 | 8.452 | <0.001 |
ice*sex (male) | 1.0 | −0.002 | 0.001 | −2.000 | 0.0450 |
Random factors | Variance explained (%) | SD | |||
Pond | 0.5 | 0.691 | |||
Date | 0.2 | 0.133 | |||
Year | 0.2 | 0.202 | |||
Model factors included: ice – ice cover on a given pond (%); IAF – the intensity of anthropogenic birds feeding conducted on a given pond; sex – sex of counted individuals; year – season, day – consecutive counting corresponding to the same weekend of a given month in different seasons; pond – given pond; * – interaction between independent variables. |
Out of all fixed effects in the best-fitted model, the IAF explained the largest percentage of the variance (Table 2). The number of overwintering Mallards increased with the IAF, and this effect was independent of the sex of individuals (Table 2, Fig. 3A). Additionally, we detected an overall increase in the number of birds with an increase in the percentage of ice cover of the ponds. Moreover, a significant effect of an interaction between sex and the percentage of ice cover indicated that this effect is sex-specific, with a larger increase in the number of individuals present in females (Table 2, Fig. 3B). Overall, males were more numerous than females in the studied area (Table 2, Fig. 3C).
Despite several factors increasing bird mortality in towns and cities (Meissner et al., 2015a; Pavisse et al., 2019; Doren et al., 2021), some species tend to select urban areas as their wintering habitats, due to higher and more stable ambient temperatures and abundant anthropogenic food resources (Luniak, 2004; Tryjanowski et al., 2015). Both these factors are important in maintaining a suitable metabolic rate crucial for the survival of an individual, which may be compromised especially in harsh wintering conditions when natural food becomes scarce and cold weather increases the energetic demand of endothermic animals. During periods of harsh wintering conditions exploiting supplementary food resources may be profitable, as it reduces time and energy spent on searching for natural food (Polańska and Meissner, 2008). Indeed, supplementary feeding in urban areas was previously accounted for causing the flocking of different bird species, as an additional source of food may also increase the carrying capacity of the wintering environment (Fuller et al., 2008; Plummer et al., 2019). Similarly, our results indicate that more intense food provisioning allows for larger numbers of overwintering Mallards to gather in a given area, independently of the size of the pond, and this effect was similar for both females and males of this species. In the seasonal environment food abundance during winter determines the distribution of overwintering species, and in some cases, it might even be one of the factors causing a norward shift in species range (Robb et al., 2008). In another Polish city, individuals ringed at low-feeding sites were more likely to relocate to more profitable feeding areas in subsequent seasons (Polakowski et al., 2010). In our study is not known whether the increase in population size was caused by local movements of individuals to the ponds with higher intensity of feeding. It is possible that such short-distance movements occur prior to longer migratory movements that were reported in Mallards as a response to a significant increase in the severity of winter (Sauter et al., 2010).
Wintering Mallards tend to move to urban areas as a response to local temperature decline, locally increasing their abundance (Meissner et al., 2015b). Here we observed a similar pattern of changes in the population size of overwintering birds, as an overall number of individuals increased with winter harshness defined as a percentage of ice cover on a given pond. Urban areas offer milder wintering conditions, with higher ambient temperatures, compared to their rural surroundings (Oke, 1982; Kalnay and Cai, 2003). This allows for birds to have lower energy expenditure for maintain their metabolic rate, without necessitating long-distance movements (Swanson and Olmstead, 1999). Additionally, for dabbing ducks, warmer urban environment may allow foraging for natural food resources, by delay the freezing over of the waterbodies. In Mallards, birds did not move to the city when unfrozen waterbodies were still available outside of the urban area (Meissner et al., 2015b). Our results point to the ice cover as a better predictor for changes in the population size of overwintering Mallards than ambient temperature. This might result from the fact that we analyzed the temperatures measured only in one meteorological station, fairly distant from the studied ponds. This approach failed to give detailed information on the mean daily temperature characteristics for each of the ponds, especially since the winter ambient temperature in the subsequent ponds increases as altitude decreases towards the seacoast (Meissner and Witkowska, 2023). Moreover, the ice cover of a given pond is a local indicator of the severity of overwintering conditions as it limits the pond's area available for ducks. Surprisingly, out of studied effects the winter harshness had a rather weak, albeit significant, impact on a number of overwintering individuals. Wintering Mallards to some extent can endure harsh winter conditions and wait out the complete freezing of waterbodies, by lowering their activity and consequently limiting their energy expenditure and food intake (Meissner and Markowska, 2009). On a continental scale, wintering Mallards more than other duck species resist freezing temperatures if the waterbody is not completely covered with ice (Sauter et al., 2010; Schummer et al., 2010). Unfortunately, we were unable to conduct our study in truly severe wintering conditions. Therefore, winter harshness was not the primary predictor of changes in the population size of overwintering Mallards, as proposed by other studies (Meissner et al., 2015b).
Male-bias sex ratio is well documented in Mallards, with the proportion of males in flocks wintering in Central Europe established at around 60% (Nilsson, 1976; Meissner and Michno, 2011). In the studied area, we observed more overwintering males than females, with an average sex ratio of 61:39. However, this ratio was not stable across studied wintering conditions, as we detected a more pronounced increase in the number of females with an increase in winter harshness. Similar results were recently shown on the whole population of Mallards overwintering in Gdańsk, where a lower share of males was present during severe wintering conditions (Meissner and Witkowska, 2023). Females of ducks could be more susceptible to lowering of the temperature, due to their smaller size and therefore higher surface to volume ratio. In other ducks, as well as other bird species with sexual size dimorphism, smaller females tend to winter in less harsh conditions present southern parts of their wintering ranges, as a way of avoiding periods of food shortage and minimizing competition with larger males (Ketterson and Nolan, 1976; Nichols and Haramis, 1980). Our results suggest that in Mallards food provisioning, together with overall higher ambient temperatures in urban areas may provide females with better wintering conditions, compared to natural wetlands in surrounding rural areas.
In this study, we demonstrated that the severity of wintering conditions promotes wintering of Mallards in an urbanized area, which was also shown in a number of studies on this species. Additionally, we provided evidence that this repose might be more pronounced in females than in males. Up until now the effect of the intensity of supplementary birds feeding on the population of wintering Mallards was mostly assumed. Although we provided a very general metric of food provisioning by the citizens, we showed that it was an important factor in shaping the abundance of the urban population of Mallards. In the studied area increased supplementing of anthropogenic food resulted in higher number of overwintering Mallards.
The authors declare that they have no known competing financial interests or personal relationships that could have appeared to influence the work reported in this paper.
Marta Witkowska: Data curation, Conceptualization, Writing – review & editing, Formal Analysis, Methodology, Visualization, Writing – original draft. Wojciech Wesołowski: Conceptualization, Data curation, Methodology, Writing – review & editing. Martyna Markiewicz: Data curation, Methodology, Visualization, Writing – review & editing. Jonasz Pakizer: Data curation, Writing – review & editing. Julia Neumann: Data curation, Writing – review & editing. Agnieszka Ożarowska: Supervision, Writing – review & editing. Włodzimierz Meissner: Conceptualization, Supervision, Writing – review & editing.
We are grateful to all members and sympathizers of the Student’s Scientific Club KOS who took part in the fieldwork. We would like to thank two anonymous Reviewers, whose comments helped improve this paper.
Supplementary data to this article can be found online at https://doi.org/10.1016/j.avrs.2024.100205.
Avilova, K.V., Eremkin, G.S., 2001. Waterfowl wintering in Moscow (1985-1999): dependence on air temperatures and the prosperity of the human population. Acta Ornithol. 36, 65-71.
|
Engler, J., Keller, M., Leszkowicz, J., Zawadzki, J., 1988. Synurbization of the mallard Anas platyrhynchos in warsaw. Acta Ornithol. 24, 9-28.
|
Hardin, J.W., Hilbe, J.M., 2018. Generalized Linear Models and Extensions, fourth ed. Stata Press, College Station, Texas.
|
Luniak, M., 2004. Synurbization - adaption of animal wildlife to urban development. In: Shaw, W., Harris, L., VanDruff, L. (Eds.), Urban Wildlife Conservation: Proceedings of the 4th International Symposium. Tucson, Arizona, p. 368.
|
Manikowska-Ślepowrońska, B., Meissner, W., 2022. Factors affecting apparent survival and resighting probability of wintering mallards Anas platyrhynchos. Ornis Fenn. 99, 83-94.
|
Meissner, W., Markowska, K., 2009. Influence of low temperatures on behaviour of mallards (Anas platyrhynchos L.). Pol. J. Ecol. 57, 799-803.
|
Meissner, W., Michno, B., 2011. Variability of the sex ratio of mallards Anas platyrhynchos wintering in the Tricity (northern Poland). In: Indykiewicz, P., Jerzak, L., Böhner, J., Kavanagh, B. (Eds.), Urban Fauna. Studies of Animal Biology, Ecology and Conservation in European Cities. UTP Bydgoszcz, pp. 415–423.
|
Meissner, W., Rowiński, P., Polakowski, M., Wilniewczyc, P., Marchowski, D., 2015b. Impact of temperature on the number of mallards, Anas platyrhynchos, wintering in cities. North-West. J. Zool. 11, 213-218.
|
Meissner, W., Witkowska, M., 2023. The effect of the temperature on local differences in the sex ratio of Mallards Anas platyrhynchos wintering in an urban habitat. Acta Oecol 119, 103900.
|
Nilsson, L., 1976. Sex-ratios of Swedish Mallard during the non-breeding season. Wildfowl 27, 91-94.
|
Oke, T.R., 1982. The energetic basis of the urban heat island. Quart. J. R. Met. Soc. 108, 551.
|
Plummer, K.E., Risely, K., Toms, M.P., Siriwardena, G.M., 2019. The composition of British bird communities is associated with long-term garden bird feeding. Nat. Commun. 10, 2088.
|
Polakowski, M., Skierczyński, M., Broniszewska, M., 2010. Effect of urbanization and feeding intensity on the distribution of wintering Mallards Anas platyrhynchos in NE Poland. Ornis Svec. 20, 76-80.
|
Polańska, H., Meissner, W., 2008. Feeding methods of mallards Anas platyrhynchos used in the city during the nonbreeding season. In: Indykiewicz, P., Jerzak, L., Barczak, T. (Eds.), Urban Fauna. Studies of Animal Biology, Ecology and Conservation in European Cities. SAR Bydgoszcz, pp. 524–529.
|
R Core Team, 2021. R: A Language and Environment for Statistical Computing.
|
Sauter, A., Korner-Nievergelt, F., Jenni, L., 2010. Evidence of climate change effects on within-winter movements of European Mallards Anas platyrhynchos. Ibis 152, 600-609.
|
Slabbekoorn, H., Ripmeester, E.A.P., 2008. Birdsong and anthropogenic noise: implications and applications for conservation. Mol. Ecol. 17, 72-83.
|
Swanson, D.L., Olmstead, K.L., 1999. Evidence for a proximate influence of winter temperature on metabolism in passerine birds. Physiol. Biochem. Zool. 72, 566-575.
|
Zhenhua Wei, Lizhi Zhou. 2023: The impact of earlier flood recession on metacommunity diversity of wintering waterbirds at shallow lakes in the middle and lower Yangtze River floodplain. Avian Research, 14(1): 100102. DOI: 10.1016/j.avrs.2023.100102 | |
Michelle García-Arroyo, Miguel A. Gómez-Martínez, Ian MacGregor-Fors. 2023: Litter buffet: On the use of trash bins by birds in six boreal urban settlements. Avian Research, 14(1): 100094. DOI: 10.1016/j.avrs.2023.100094 | |
Cristel Álvarez-Castillo, Ian MacGregor-Fors, Stefan L. Arriaga-Weiss, Claudio Mota-Vargas, Diego Santiago-Alarcon. 2022: Abundance of White-fronted Parrots and diet of an urban parrot assemblage (Aves: Psittaciformes) in a green Neotropical city. Avian Research, 13(1): 100019. DOI: 10.1016/j.avrs.2022.100019 | |
Joshua M. Diamond, Michael S. Ross. 2019: Exotic parrots breeding in urban tree cavities: nesting requirements, geographic distribution, and potential impacts on cavity nesting birds in southeast Florida. Avian Research, 10(1): 39. DOI: 10.1186/s40657-019-0176-3 | |
Kang Luo, Zhaolu Wu, Haotian Bai, Zijiang Wang. 2019: Bird diversity and waterbird habitat preferences in relation to wetland restoration at Dianchi Lake, south-west China. Avian Research, 10(1): 21. DOI: 10.1186/s40657-019-0162-9 | |
Xiaodan Wang, Fenliang Kuang, Kun Tan, Zhijun Ma. 2018: Population trends, threats, and conservation recommendations for waterbirds in China. Avian Research, 9(1): 14. DOI: 10.1186/s40657-018-0106-9 | |
Andrew J. Hamilton, Chloé Conort, Aurore Bueno, Christopher G. Murray, James R. Grove. 2017: Waterbird use of farm dams in south-eastern Australia: abundance and influence of biophysical and landscape characteristics. Avian Research, 8(1): 2. DOI: 10.1186/s40657-016-0058-x | |
K. M. Aarif, Aymen Nefla, S. B. Muzaffar, K. K. Musammilu, P. K. Prasadan. 2017: Traditional fishing activities enhance the abundance of selected waterbird species in a wetland in India. Avian Research, 8(1): 16. DOI: 10.1186/s40657-017-0073-6 | |
China Coastal Waterbird Census Group, Qingquan Bai, Jianzhong Chen, Zhihong Chen, Guotai Dong, Jiangtian Dong, Wenxiao Dong, Wing Kan Vivian Fu, Yongxiang Han, Gang Lu, Jing Li, Yang Liu, Zhi Lin, Derong Meng, Jonathan Martinez, Guanghui Ni, Kai Shan, Renjie Sun, Suixing Tian, Fengqin Wang, Zhiwei Xu, Yat-tung Yu, Jin Yang, Zhidong Yang, Lin Zhang, Ming Zhang, Xiangwu Zeng. 2015: Identification of coastal wetlands of international importance for waterbirds: a review of China Coastal Waterbird Surveys 2005-2013. Avian Research, 6(1): 12. DOI: 10.1186/s40657-015-0021-2 | |
Guogang ZHANG, Wei LIANG, Dongping LIU, Fawen QIAN, Yunqiu HOU, Wenba SU, Kilburn MIKe, Holmes JEMI, Kwok Shing LEE. 2010: Species abundance and conservation of coastal wintering waterbirds in Hainan Island, China. Avian Research, 1(3): 204-210. DOI: 10.5122/cbirds.2010.0014 |
Conditional model | R2 cond. | R2 marg. | AiCc | ΔAICc | wi | df. resid | Dev | |
Reduced (with ice) | N = ice + IAF + sex + ice * sex + r (year) + r (day) + r (pond) | 0.54 | 0.27 | 5514.7 | 0 | 0.669 | 894 | 5495.7 |
Global (with ice) | N = ice + IAF + sex + size + ice ∗ sex + IAF ∗ sex + r (year) + r (day) + r (pond) | 0.53 | 0.28 | 5517.3 | 2.6 | 0.331 | 892 | 5493.0 |
Reduced (with temp | N = temp + IAF + sex + r (year) + r (day) + r (pond) | 0.52 | 0.25 | 5701.9 | 187.2 | <0.001 | 927 | 5683.8 |
Global (with temp) | N = temp + IAF + sex + size + temp ∗ sex + IAF ∗ sex + r (year) + r (day) + r (pond) | 0.52 | 0.28 | 5702.6 | 187.9 | <0.001 | 924 | 5678.3 |
Null | N = r (year) + r (day) + r (pond) | 0.50 | 0 | 5798.2 | 283.5 | <0.001 | 930 | 5786.2 |
Model factors included: ice – ice cover on a given pond (%); temp – ambient temperature (℃); IAF – the intensity of anthropogenic birds feeding conducted on a given pond; sex – sex of counted individuals; size – size of given pond (m2); year - season, day – consecutive counting corresponding to the same weekend of a given month in different seasons; pond – given pond; r () – random factor; * – interaction between independent variables; Provided metrics: R2 cond. – conditional R2, R2 marg. – marginal R2; AICc – Akaike’s Information Criterion for small sample size; ΔAICc – the difference between AICc of a given model and the model with the lowest AICc; df. resid – residual degrees of freedom; Dev – deviance. |
Fixed effects | Variance explained (%) | Estimate | SE | z-value | p-value |
Zero-inflation model | |||||
Intercept | −3.214 | 0.232 | −13.86 | <0.001 | |
Conditional model | |||||
Intercept | 1.129 | 0.268 | 4.208 | <0.001 | |
IAF | 22.5 | 0.068 | 0.018 | 3.660 | <0.001 |
Ice | 1.0 | 0.002 | 0.001 | 2.658 | 0.008 |
sex (male) | 1.8 | 0.468 | 0.055 | 8.452 | <0.001 |
ice*sex (male) | 1.0 | −0.002 | 0.001 | −2.000 | 0.0450 |
Random factors | Variance explained (%) | SD | |||
Pond | 0.5 | 0.691 | |||
Date | 0.2 | 0.133 | |||
Year | 0.2 | 0.202 | |||
Model factors included: ice – ice cover on a given pond (%); IAF – the intensity of anthropogenic birds feeding conducted on a given pond; sex – sex of counted individuals; year – season, day – consecutive counting corresponding to the same weekend of a given month in different seasons; pond – given pond; * – interaction between independent variables. |