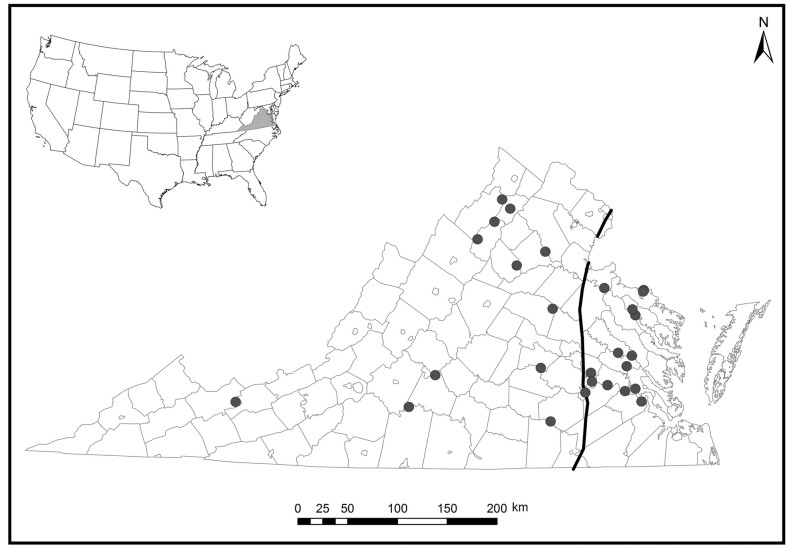
Citation: | Flavio Monti, Luca Nelli, Carlo Catoni, Giacomo Dell'Omo. 2019: Nest box selection and reproduction of European Rollers in Central Italy: a 7-year study. Avian Research, 10(1): 13. DOI: 10.1186/s40657-019-0150-0 |
Changes and increased mechanisation of agricultural practices have influenced the biodiversity composition of farmland habitats and caused a decline of bird communities in many European countries. The removal of shrubs and large trees rich in natural cavities, has also led to a drastic decrease in nest site availability for cavity-nesting bird species. Nest-boxes are a common conservation tool used to improve nest-site availability, and have helped to reverse declines in many endangered bird populations. Nonetheless to maximize the results of such interventions it is crucial to know where nest-boxes should be sited. The objective of this study was to investigate the effectiveness of the nest-box program for the European Roller (Coracias garrulus) population of Lazio region (Central Italy). More specifically, we focused on what landscape features were preferred (or avoided) in the process of nest box selection and how they influenced population's breeding parameters. Particular attention was paid to identifying potential limitations and to provide management recommendations for future interventions.
Using data from 70 nest boxes sited on power lines monitored over a 7-year period (representing 140 breeding attempts), we developed probability functions to evaluate if nest box location, in terms of distance from habitat resources and habitat composition and structure, had an effect on nest box occupancy and on the main reproductive parameters.
Nest boxes were more likely to be occupied if they were located near arable fields and in areas characterized by a higher amount of incoming solar radiation. Higher fledging success was associated with fallow fields and with a moderate/low habitat structural complexity. Higher breeding success was associated with solar radiation and with greater distance from urban areas.
Our results highlight the importance of specific habitat variables in influencing nest occupancy, and show which drivers primarily affect species' reproduction and persistence over time. Siting nest boxes in habitats where occupancy rate and fledging success is higher, such as in arable and fallow fields and on south-facing slopes where solar radiation is maximised, may help to extend the suitable habitat for rollers and facilitate its local expansion.
It is well known that the neurotoxic properties of mercury (Hg) and methylmercury (MeHg) represent a substantial risk to wildlife, in particular, higher trophic level species (Scheuhammer 1987; Yates et al. 2004, 2005; Evers et al. 2005; Hopkins et al. 2013). Wolfe et al. (1998) suggested that the impacts of elevated Hg are likely more significant when the individuals are in the developmental stages of growth. Moreover, elemental Hg readily methylates into its more toxic form given appropriate environmental conditions (sulfide and dissolved organic matter) (Miller et al. 2007), making it available to wildlife and humans (Thompson 1996; Evers et al. 2005; Kramar et al. 2005). The widespread transport of Hg via atmospheric processes, and subsequent atmospheric deposition, ensures that Hg will remain globally ubiquitous (Wiener et al. 2003). Much of the available Hg in the atmosphere is due, in large part, to a myriad of industrial sources such as chlor-alkali facilities (Maserti and Ferrara 1991), as well as the continued combustion of coal and other fossil fuels (Berlin et al. 2007). Due to extensive risks posed to humans and wildlife from the accumulation of Hg, every state within the continental US has fish consumption advisories already in place.
Research on Hg in Bald Eagles (Haliaeetus leucocephalus) has been conducted in numerous eastern states including South Carolina (Jagoe et al. 2002), Florida (Wood et al. 1996), Maine (Welch 1994), New York (DeSorbo et al. 2008, 2009), and the Great Lakes region of the US (Bowerman et al. 1994). Although the Bald Eagle has been studied extensively in the Coastal Plain of Virginia (Buehler 1990; Buehler et al. 1991; Watts et al. 2011), little research on Bald Eagles has been conducted in the inland regions, due in part to a lack of knowledge of the inland distribution. Wiemeyer et al. (1984) analyzed non-viable eggs from Bald Eagles in the Chesapeake Bay, and reported concentrations of Hg between 0.03 and 0.17 mg/kg, and Cristol et al. (2012) found that eagles residing near the Chesapeake Bay exhibited low concentrations of Hg in molted feathers. In contrast to the Chesapeake Bay, several inland areas in the state have been identified as hotspots for Hg exposure to other avian species, including the Shenandoah River (Jackson et al. 2011; Cristol et al. 2012; Hopkins et al. 2013) and the North Fork of the Holsten River (Echols et al. 2009). Jackson et al. (2011) noted that forest songbirds were exhibiting elevated Hg concentrations over 130 km downstream of the known point source on the Shenandoah River, with Hg distributed in downstream habitat features such as flood plain areas.
Within the state of Virginia, the majority of the known eagle population (n = 726 occupied territories 2011) nests within the coastal plain associated with the Chesapeake Bay and the major tributaries that feed the bay (Watts et al. 2011). However, more than 25% of the total population (n > 200) nests in the Piedmont and Mountain regions (Kramar D., unpublished data). Prior to 2007, little information regarding the inland population was available. As elevated Hg in wildlife has been positively associated with freshwater environments, we expect that Hg concentrations in central and western Virginia eagles would exhibit significantly higher levels in blood and feather than those of the coastal plain. Moreover, as Hg accumulates in the body with age (Kamman et al. 2005), nestling eagles that are expressing levels at or above those considered sub-lethal are an important indicator of Hg in the immature and adult cohorts of the same eagle population.
Our objectives were to establish reference levels for Hg contaminants in Bald Eagles in coastal Virginia based on previous work (Cristol et al. 2012) and from collection and analysis of new tissue samples from nestling Bald Eagles. This allowed us to test our hypothesis that inland Hg concentrations were significantly higher than the Hg concentrations found in Bald Eagles in the coastal population. We also evaluated the spatial variability of Hg across the inland population, and analyzed the relationship between blood Hg and feather Hg.
We conducted this throughout the state of Virginia between the spring of 2007 and the spring of 2012. The population of eagles in Virginia has rebounded from a low of approximately 80 pairs in the 1970s (Abbott 1978), to over 800 by 2012 for both the coastal and inland populations (Watts et al. 2011; Kramar D., unpublished data). We sampled 28 nests across the state, and 46 individuals (Fig. 1). In instances where there were multiple individuals per nest, we used the average Hg concentration between the individuals to represent the Hg concentration per nest. We also checked to see if using either the minimum or maximum values had any effect on the resulting models. Coastal nests were defined as those that fell within the coastal plain (east of the fault line), and inland nests were defined as those that fell within the Piedmont and Mountain physiographic regions (west of the fault line). Nests were randomly selected and assessed for climbability based on the condition of the tree, and the age of the individuals. In a small number of cases, selected nests could not be climbed due to lack of approval from the property owner. In these cases, we selected another nest.
We collected samples from 4 to 7 week old eaglets by climbing to the nests and lowering them to the ground in a protective bag. We collected blood samples from the brachial vein in the wing using 21 gauge butterfly needles and 4 cc lithium heparinized vacutainers. Each vacutainer was labeled with a unique nest identification number and the federal band number, and the blood was packed on ice and frozen within 4 h of collection. We collected two to three contour feathers from the breast of each individual. The samples were placed in a brown envelope, sealed, and labeled with the nest identification number and federal band number. All activities were conducted under the appropriate state and federal permits.
Samples were analyzed using a Milestone DMA-80 Direct Mercury Analyzer (DMA) at the Sawyer Environmental Research Center, located at the University of Maine, Orono. Analysis of samples was conducted after calibrating the DMA using a certified standard Hg solution (SPEX CertiPrep) (Cell 1: R2 = 1.0000, Cell 2: R2 = 0.9998). All calibration and sampling consisted of measuring both the SPEX and the samples to the nearest 0.0001 g using a Metler Toledo digital scale, Model AT201). Quality assurance measures included the use of standard reference materials. In addition to the standard reference materials, two system and method blanks, one 10-ng prepared Spex sample, one 60-ng prepared Spex sample, one replicate, and one matrix spike were run as quality assurance per every ten biological samples. Feathers were mechanically washed following Ackerman et al. (2007) in a 1% Alconox solution, rinsed 6 times in deionized water, and dried for 24-48 h. The recovery of standard reference materials was near 100% and well within the accepted range of 90-110%. QC verification was 105.6% and 103.1% during calibration. Similarly, replicate samples were well within accepted parameters. Spiked samples were based on the addition of 0.05 g of 10 ng SPEX. Once prepared, samples were weighed out and placed in quartz or nickel boats for analysis (depending on the matrix type: quartz = blood, nickel = feather). No more than 20 samples were analyzed at any one time, with QC procedures conducted following every ten samples. Analysis generally followed US Environmental Protection Agency Method 7473 (USEPA 2000).
We conducted a power analysis both prior to collection of samples and post sampling, to insure that the sample size was adequate to make inferences regarding the population. Given a standard deviation across all samples of 0.205, and 28 samples (individual nests), the power to determine differences between the coastal and inland populations at α = 0.25 was 0.874.
We initially log-transformed the Hg scores and applied the Shapiro Wilks Goodness of Fit test to determine whether they met normality requirements. Because the log-transformed data did not approximate a normal distribution, we compared the Hg in coastal and inland populations using the non-parametric Wilcoxon test. To determine the effect of point contaminant sources on the Shenandoah River, we ran a second Wilcoxon test while withholding the data from the Shenandoah River.
To test for a relationship between blood Hg and feather Hg, we developed a predictive model of the function. We initially fit a linear regression model and evaluated the fit by analyzing the residual distribution. Due to the non-linear nature of our sample distribution we utilized a non-linear quadratic regression to appropriately model the relationship.
The mean blood Hg concentration across Virginia eagles was x = 0.180 mg/kg, SE = 0.036. The mean concentration for feather Hg was x = 5.024 mg/kg, SE = 0.842. The lowest blood Hg concentrations sampled (0.012 mg/kg, SE = 0.012) were located in birds from the coastal plain of Virginia, specifically on the Pamunkey River. The highest concentrations were sampled in birds located inland on the Shenandoah River, in particular on the South Fork of the Shenandoah River (0.974 mg/kg, SE = 0.065).
Blood Hg concentrations in the coastal plain (x = 0.06 mg/kg, SE = 0.012 mg/kg, range = 0.01-0.15 mg/kg) were significantly less (Z = 4.676, p < 0.0001, χ2 = 21.96, p < 0.0001) than blood Hg concentrations in inland nests (x = 0.324 mg/kg, SE = 0.065 mg/kg, range = 0.06-0.97 mg/kg). Feather Hg concentrations in the coastal plain (x = 2.16 mg/kg, SE = 0.420 mg/kg, range = 0.62-9.998 mg/kg) were significantly less (Z = 4.168, p < 0.0001, χ2 = 17.465, p ≤ 0.0001) than feather Hg concentrations in inland nests (x = 8.43 mg/kg, SE = 1.47, range = 3.81-21.14 mg/kg). After removing the Shenandoah River from the analysis, we still found that the coastal blood Hg concentrations were significantly less (Z = 4.0, p < 0.0001, χ2 = 16.08, p < 0.0001) than blood Hg concentrations in the inland nests. Likewise, after removing the Shenandoah River from the analysis, feather Hg concentrations from the coastal plain were significantly less (Z = 3.38, p = 0.0007, χ2 = 11.52, p = 0.0007) than feather Hg concentrations from the inland nests.
The quadratic regression model provided the best overall fit to our data (Fig. 2). This cumulative function (Fig. 2) supported the prediction that Hg in feathers accumulated over time, representing total body burden, while Hg in blood represented recent dietary uptake. In that respect, the relationship between feather and blood Hg, in this study, is a cumulative function. The analysis of variance table indicated a sufficient model fit (F = 12.97, df = 1, SS = 0.133, p = 0.001) that represents 83.4% of the variance of blood Hg concentrations.
While we found that the coastal population of nestling Bald Eagles in Virginia had Hg concentrations that are not of a level currently of concern, the inland population has Hg burdens that are at significantly higher concentrations than previously expected. These findings support Cristol et al. (2012) who also documented low levels of Hg in Bald Eagles in coastal Virginia. To establish a threshold for what may be considered sub-lethal concentrations of Hg, we used a blood Hg concentration of 0.70 mg/kg (Desorbo and Evers 2007). Whereas Bald Eagles along several inland rivers exhibited blood Hg levels approaching that threshold, only the Shenandoah River exceeded it (Fig. 3). No eagles along rivers in coastal Virginia approached this threshold. Specifically, rivers where eagles exhibited elevated concentrations include the inland portions of the Rappahannock River, Nottoway River, James River, and Wolf Creek. The higher concentrations of feather Hg found in this study, not surprisingly, follow patterns similar to that of the blood Hg concentrations (Fig. 4).
Given that Hg is sequestered rapidly during feather growth, it is not unreasonable to expect that once feather development is complete, levels of blood Hg would increase. Condon and Cristol (2009) suggest that the most likely period for Hg to approach sub-lethal levels is immediately following fledging, when feathers are no longer sequestering Hg from the blood. Thus an individual at 4 weeks of age, which is approaching a 0.70 mg/kg threshold, would likely exceed that threshold once the feathers have completely developed. This relationship between blood Hg and feather growth in Bald Eagles identifies the need for additional sampling of adult Bald Eagles in inland Virginia. Moreover, additional research should be conducted on the North Fork of the Shenandoah River as Hg concentrations here were also approaching the 0.70 mg/kg threshold.
Inland eagle populations within Virginia are exposed to a greater amount of Hg than their coastal counterparts. This variation in Hg concentrations is likely due to a number of different factors. First, Hg methylates more readily in freshwater environments, and as such, that Hg would be available to wildlife that reside there. Second, we speculate that the elevated concentrations of Hg in inland eagle populations are also influenced by the variation in potential prey between the freshwater and saline environments. Because of these factors, the freshwater environment of the Piedmont and Mountain regions warrants continued monitoring to understand the long-term implications of Hg in these areas. In particular, additional work along the Nottoway River, Holsten River, Shenandoah River, Rappahannock River, and Wolf Creek should be conducted.
From a management perspective, additional efforts to facilitate remediation and further understand the full distribution and potential implications of Hg within the inland portions of the state should be considered. These efforts should include research into potential point sources on the above mentioned rivers, as well as further research into the Hg concentrations in adult Bald Eagles. Lastly, given the Hg concentrations found on the rivers sampled in this research, additional efforts should be focused on sampling rivers that were not represented such as the Powell River, the Holston River, and the New River.
DEK collected and analyzed the data associated with this work. BC aided in editorial suggestions, geospatial analysis, and presentation of material. SP assisted in analysis, editorial suggestions, and geospatial analysis. JC assisted in editorial comments and statistical analysis. All authors read and approved the final manuscript.
We would like to extend our sincere gratitude to the Virginia Department of Game and Inland Fisheries: specifically Sergio Harding and Jeff Cooper. In addition, we would like to thank the staff at the Wildlife Center of Virginia, Bryan Watts and Libby Mojica from the University of William and Mary, and Justin Miller, Kari McMullen, Emily Wright, and Jessica Rich for extensive field assistance. Samples were collected under federal permits held by the University of William and Mary (Dr. Bryan Watts) or the Virginia Dept. of Game and Inland Fisheries (Jeff Cooper), and state threatened and endangered species permits. IACUC approval was acquired through the Virginia Tech Institutional Animal Care and Use Committee.
The authors declare they have no competing interests.
The datasets generated during and/or analyzed during this study are available from the corresponding author on reasonable request.
Not applicable.
IACUC approval was secured through Virginia Polytechnic Institute and State University for the duration of the project.
1. | Nicolantonio Agostini, Gianpasquale Chiatante, Giacomo Dell’Omo, et al. Differential autumn migration between sex and age groups in the Western marsh harrier: a longitudinal pattern analysis. Ethology Ecology & Evolution, 2021, 33(1): 73. DOI:10.1080/03949370.2020.1820581 |
2. | Jesús Nadal, Carolina Ponz, Antoni Margalida. The end of primary moult as an indicator of global warming effects in the Red-legged Partridge Alectoris rufa, a medium sized, sedentary species. Ecological Indicators, 2021, 122: 107287. DOI:10.1016/j.ecolind.2020.107287 |
3. | Oluwadunsin E. Adekola, David G. Allan, Zephne Bernitz, et al. Extent and symmetry of tail moult in Amur Falcons. Journal of Ornithology, 2021. DOI:10.1007/s10336-021-01874-0 |