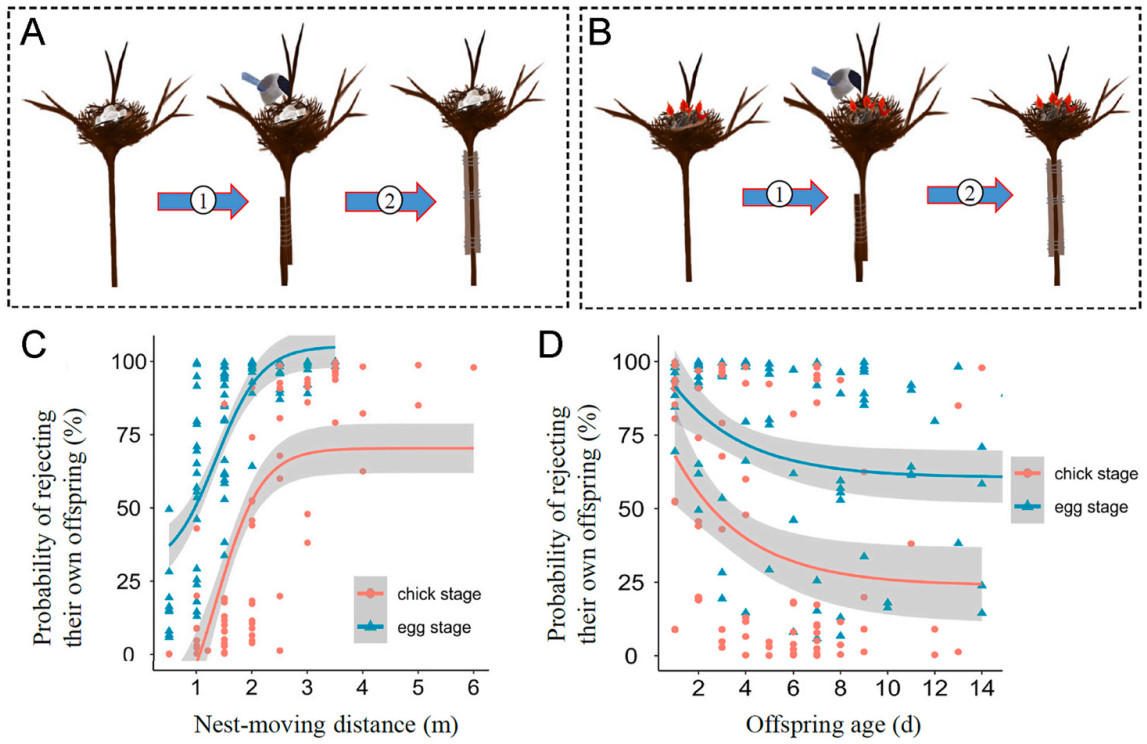
Citation: | Hong Jin, Qi Xia, Ziqing Gao, Yujie XuanYuan, Beibei Dong, Ming Li, Qingjian Liang, Jinsong Liu. 2025: Decreased basal thermogenesis is associated with the downregulation of cellular metabolic activity of organs and serum thyroid hormones in fasting Eurasian Tree Sparrows (Passer montanus). Avian Research, 16(1): 100220. DOI: 10.1016/j.avrs.2024.100220 |
Food is a critical environmental factor that influences animal survival, especially for small passerines due to their high mass-specific metabolic rates. Basal metabolic rate (BMR) reflects the energy expended by endothermic animals for basic physiological processes and constitutes a major part of their daily energy budget. Some birds have been shown to employ compensatory mechanisms during food shortages, temporarily reducing these self-maintenance expenditures without using hypothermia. However, the mechanisms of BMR adjustment remain unexplored. In the present study, we assessed the phenotypic variation in basal thermogenesis of Eurasian Tree Sparrows (Passer montanus) by comparing a control group to groups fasted for 6, 12, 18, and 24 h. We focused on the correlation between a reduction in energy metabolism and the alterations of cellular metabolic activities, mitochondrial substrate supply, and changes in serum thyroid hormones during fasting. Our data indicated that fasting groups had significantly lower body mass, BMR, body temperature, and body fat content. Furthermore, fasting groups had significantly lower glycogen levels, mitochondrial state 4 respiration and cytochrome c oxidase (CCO) activity in the liver, and CCO activity in pectoral muscle. The levels of avian uncoupling protein (av-UCP) mRNA were significantly reduced, while the levels of myostatin protein in pectoral muscle were significantly increased in the fasting groups. Furthermore, the groups subjected to fasting exhibited significantly lower levels of serum glucose, triglyceride, thyroxine (T4), and triiodothyronine (T3). Positive correlations were observed between the following pairs of variables: log BMR and log body mass, log body mass and log body fat, log BMR and log state 4 respiration in the liver, log BMR and log CCO activity in the liver and muscle, log BMR and log av-UCP mRNA expression, whereas a negative correlation was observed between log BMR and log myostatin level. In addition, a positive correlation was also detected between log T3 and each of the following: log BMR, state 4 respiration, and log CCO activity in the liver. Our results suggested that decreased metabolic thermogenesis via down-regulation in cellular aerobic capacity of organs and serum thyroid hormones may be an important survival strategy for fasting Tree Sparrows to reduce energy expenditure.
The theory of inclusive fitness highlights the significance of kinship as a fundamental explanation for the occurrence of social behaviors (Hamilton, 1964). Regarding the evolution of parental care, parenting behaviors directed towards their own offspring have undergone positive selection and should have been favored by natural selection (Royle et al., 2012). However, alternative parenting behaviors, such as rejecting their own offspring and accepting unrelated offspring, are widely observed across various animal species and life-history stages (Trivers, 1974). For example, in cases of extra-pair copulations (EPCs), cuckolded males may abandon the mixed brood, including their own offspring (Møller and Birkhead, 1993; Griffith et al., 2022; Perlut et al., 2012). In cases of brood parasitism or semi-parasitism, host parents often provision the parasitic young in their nests (Sorenson and Payne, 2002; Bouwman et al., 2005; Du et al., 2015). Obviously, both alternative parenting behaviors will impose negative effects on parental fitness (Hamilton, 1964). To demonstrate the prevalence of alternative parenting behaviors across animal species, it need to identify the potential effect of offspring recognition capacity on parental care behaviors, as well as potential factors facilitating the occurrence of alternative parenting behaviors.
Parental capacity for offspring recognition can influence their tradeoffs between rejecting and accepting a young. For instance, in the cases of EPCs, parental care strategies of cuckolded males depend largely on whether they can distinguish their own offspring from the extra-pair young. If they are able to recognize their own offspring, the optimal parenting behavior will be selectively providing care for their own offspring. They provision extra-pair young only when the presence of unrelated young is beneficial to their offspring, such as maintaining thermal stability in the nest (Smith et al., 2018). If cuckolded males are unable to recognize their own offspring, provisioning unrelated young occurs only when they have sired the majority of mixed paternity and the benefits of provisioning unrelated young outweigh the cost of abandoning the current brood (García-Vigón et al., 2009; Du et al., 2015; Gao et al., 2020). Otherwise, cuckolded males will probably reduce paternal care for the mixed brood (Møller and Birkhead, 1993; Perlut et al., 2012). Therefore, it is imperative to ascertain the parental capacity for offspring recognition as a prerequisite for demonstrating the occurrence of alternative parenting behaviors.
Parental capacity for offspring recognition is closely associated with whether offspring can give out an individualized, fixed signal (Dowling et al., 2016). It has been reported in vertebrates that various phenotypic traits have the potential as the recognition cue, including acoustic features (Malmkvist, 2019), facial plumage (Johnson and Freedberg, 2014), olfactory odor (Mateo, 2017), and chemical fingerprints (Pitcher et al., 2015). These traits change remarkably with offspring growth (Mak and Weiss, 2010; Johnson and Freedberg, 2014). The ontogeny of offspring phenotypic traits indicates that parents depend heavily on an early association with their offspring so that they can learn the developmental status of offspring traits (Luther, 2008). In this scenario, any factors that disrupt parent–offspring association or introduce unrelated young into the nest before offspring phenotypic traits fully develop can impact parental capacity for offspring recognition. It is crucial to consider this influence when demonstrating the occurrence of alternative parenting behaviors.
In order to investigate the factors prompting the occurrence of alternative parenting behaviors, we conducted a series of experiments by manipulating the nest and offspring signals in a Tibetan population of the Azure-winged Magpie (Cyanopica cyanus). EPCs occur frequently in the Tibetan Azure-winged Magpies and cuckolded males do not reduce but instead continuously provide parental care for the mixed brood (Gao et al., 2020a). The indiscrimination of Azure-winged Magpies in providing parental care for their own offspring and unrelated young provides us a good system to address the role of parental capacity of offspring recognition in determining their parental care strategies.
In this study, three types of experiments were designed to examine parental reactions to the manipulated offspring. In the nest resettlement experiment, we manipulated only the nest spatial position to test whether nest-moving distance and offspring age influenced the occurrence of parental rejecting their own offspring. In the cross-fostering experiment, we exchanged the entire brood between two nests to test whether the changed number and age of offspring influenced the occurrence of parental accepting unrelated young. In the nest duplication experiment, we provided parents a choice between familiar offspring and unfamiliar unrelated chicks, as well as that between unfamiliar offspring and familiar unrelated chicks, which enabled us to verify the effects of time depriving parent–offspring association on the occurrence of both alternative parenting behaviors.
This study was conducted in Luqu County, Gansu Province of China (34.6°N, 102.5° E, elevation 3400 m a.s.l.), from 2012 to 2021. The typical landscape of this region is alpine meadow, with clusters of shrubs, such as Berberis hemsleyana, Hippophae rhamnoides and Salix caprea, dispersed along the bank of the Tao River. Azure-winged Magpies constructed their nests mainly in the shrubs of H. rhamnoides and S. caprea. Constrained by their preference for shrub-nesting, the Tibetan population comprised of a string of colonies. In each colony, breeders nested in a highly-clumped pattern (20–180 nests/ha; Ren et al., 2016). As summer residents of the Tibetan Plateau, Azure-winged Magpies overwinter in the low-altitude regions, and migrate to the high-altitude habitat to breed. Experienced breeders usually returned to their previous colony and selected nest-sites near their territory from the previous year.
Life-history data collection was carried out every year according to Ren et al. (2016). Eggs and nestlings were marked according to their laying/hatching orders, and measured every 1–3 days until they fledged or disappeared because of nest predation or starvation. Parenting behaviors of provisioning nestlings in the nest were recorded using a digital camcorder (ZX1; Eastman Kodak Company), which as fixed 1 m above the nest ever since adults were captured and banded to allow individual identification (Da et al., 2018). Video recordings for each nest lasted for at least three discontinuous days. Data on parental behaviors were extracted from the videos to determine whether parents in a focus nest provided parental care and which chicks were provisioned (Zhu et al., 2023).
Three experiments were designed, namely nest resettlement, cross-fostering and nest duplication. In the nest resettlement experiment, a nest was manipulated by vertically moving it 0.5–6 m downwards to a new position. The branch supporting the nest was carefully severed and lowered to an appropriate height before being reattached to the remaining branch using sticks and metal wire upon the completion of the experiment (Fig. 1). To facilitate ease of manipulation, only nests situated on vertical stems of shrubs at heights ranging from 6 to 8 m above ground level were selected. Depending on the precise location of each nest along the stem, we ensured that its new position maintained a minimum distance of 2 m from ground level. The nest-moving and resettling procedure was completed in 5 min on sunny days to reduce the potential effect of low temperature on the offspring. Then, parental behaviors were monitored in subsequent half an hour. As Azure-winged Magpies always returned to their nests in less than 10 min during we checked the nest content, half an hour is enough for us to determine whether parents rejected their own offspring or not. Behavioral observations indicated that parents in all the experiments had returned to the manipulated nest: if parents did not resume their interrupted parenting behaviors, they were considered to reject their own offspring; if parents continued to incubate eggs or provision chicks, they were considered to have the capacity for offspring recognition. To exclude the possibility that parents rejected their own offspring because of the interference caused by the nest-moving protocol, the cut branch was reinstated to its original position after the experiment was ended, parental responses in the reinstated nest were recorded and used as a control. Behavioral observations indicated that parents in all the experiments had returned to the reinstated nest and resumed their parenting behaviors after the experiment, implying that the nest-moving protocol was not the reason leading some parents to reject their own offspring. In this study, the experiment was repeated in 96 nests at the egg stage (Fig. 1A) and in 84 nests at the chick stage (Fig. 1B), respectively.
In the cross-fostering experiment, all offspring between two nests were cross-fostered in three forms, both at the egg stage, both at the chick stage, or one at the egg stage and the other at the chick stage (Fig. 2A–C), without controlling for the number and age of offspring. This experiment facilitated us to calculate the probability of parents providing care for unrelated young because only foreign offspring were provided to adults. If parents returned to the manipulated nest and incubated foreign eggs or provisioned foreign chicks in subsequent half an hour, they were considered to accept unrelated young; if parents returned to the manipulated nest but did not incubate foreign eggs or provisioned foreign chicks, they were considered to have the capacity for offspring recognition. In this study, we cross-fostered a total of 23 pairs of nests both at the egg stage, 27 pairs of nests both at the chick stage, and 25 pairs of nests at the egg and chick stage.
In the nest duplication experiment, an artificial nest was first constructed using an abandoned nest cup and fixed it to a new position with 0.5 m distance from a focus nest (hereafter referred to duplicated and original nest). According to the results of the nest-resettlement experiment, where parents consistently provided parental care for their offspring in all instances when the nest was relocated by 0.5 m, we ensured that the distance of 0.5 m between the duplicated nest and the original nest site did not influence whether parents provisioned or refused to provision chicks in the duplicated nest. Thereafter, two kinds of procedures were carried out. The first provided parents a choice between familiar offspring and unfamiliar unrelated chicks. During the experiment, all chicks in the original nest were transferred to the duplicated nest, and then same-aged chicks from another nest were transferred to the original nest (Fig. 3A). In this study, we successfully carried out 96 replicates for the first nest duplication experiment that covered various nestling ages. The second provided parents a choice between unfamiliar offspring and familiar unrelated chicks. Originally, new hatchlings in the original nest were cross-fostered with the same-aged hatchlings in another nest so that all chicks were raised by their foster parents. After they were 11 days old, one or two familiar, unrelated chicks were transferred to the duplicated nest, and the same-numbered unfamiliar offspring were transferred to the original nest (Fig. 3B). Such a design could exclude the possibility that parents would inevitably reject some chicks if there were too many chicks begged simultaneously in one provisioning process. To minimize the effect of brood-mates' begging on parental selection, only fostered chicks were kept in the original and duplicated nests, and their brood-mates were temporarily raised in an artificial nest during the experiment. In this study, we successfully carried out 16 replicates for the second nest duplication experiment that used only 11-day-old chicks. Parental behaviors were monitored by the way described above in subsequent an hour of transferring the chicks. In the first nest duplication experiment, if parents only provisioned the chicks in the duplicated nest, they were considered to have the ability of recognizing their offspring; if parents provisioned the chicks in the original nest, they were considered to accept unrelated young, irrespective of whether they provisioned the chicks in the duplicated nest. In the second nest duplication experiment, if parents provisioned the unfamiliar offspring in the original nest, they were considered to have the capacity for offspring recognition; if parents provisioned the familiar unrelated chicks in the duplicated nest but not the unfamiliar offspring in the original nest, they were considered to make both errors. By simultaneously providing genetic offspring and unrelated chicks in the original and duplicated nest, this experiment facilitated us to determine whether parents have ability of recognizing their offspring.
Generalized linear models (GLMs) were fitted to test factors that might influence whether parents returned and resumed their interrupted parenting behaviors in the manipulated nest (binomial distribution, with logit link function). In the nest resettlement experiment, predictor variables included the age of offspring (i.e., the incubation days for eggs, and the hatching days for chicks), nest-moving distance, and the breeding stage when the experiment was carried out (1 = at the egg stage, 0 = at the chick stage). In the cross-fostering experiment between two nests at the egg stage, predictor variables included the changed number of eggs and incubation days. In the cross-fostering experiment between two nests both at the chick stage, predictor variables included the age of parents' own offspring, and the age difference between cross-fostered chicks. In the cross-fostering experiment between two nests of eggs and chicks, predictor variables included the number of offspring, the changed number of offspring, and the age of offspring. In the first nest duplication experiment, predictor variables included the number and age of chicks. In the second nest duplication experiment, the frequency of parents provisioning familiar unrelated young and unfamiliar offspring were compared using chi-square test. During the fitting of GLMs, the predicted probability of parents rejecting their own offspring or providing parental care for unrelated young in the experiment was restored as a new variable and then regressed against the independent variable with significant effect on the dependent variable.
All statistical analyses were carried out using R (Version 3.4.4). Descriptive results are presented as mean ± standard effort of the mean (SEM). The null hypothesis, positing an equal probability for parents to return and resume their interrupted parenting behaviors in a manipulated nest, will be rejected when the two-tailed P-value is less than 0.05.
In the nest resettlement experiments (Fig. 1), 27.37% (26/95) of incubation parents and 58.33% (49/84) of provisioning parents returned to the manipulated nests and resumed their interrupted parenting behaviors. In the control, all parents returned to the reinstated nests and resumed their parenting behaviors. Whether parents resumed their interrupted parenting behaviors in a manipulated nest was significantly related to the nest-moving distance and offspring age, and it differed significantly between at the egg and chick stage (Table 1). The larger the distance of nest-moving (Fig. 1C) and the younger the offspring (Fig. 1D), the higher the probability that parents rejected their own offspring. The probability of parents rejecting their own offspring was higher at the egg stage than at the chick stage (Fig. 1C and D).
Predictor variables | β ± SE | n | z | P | |||||||||||||||
Intercept | 3.30 ± 1.75 | 179 | 1.88 | 0.06 | |||||||||||||||
Offspring age (day) | 0.34 ± 0.08 | 179 | 4.36 | < 0.001 | |||||||||||||||
Nest-moving distance (m) | −2.44 ± 0.39 | 179 | −6.22 | < 0.001 | |||||||||||||||
At the chick stagea | 4.91 ± 0.82 | 84 | 6.01 | < 0.001 | |||||||||||||||
aNote: the value at the egg stage was set as the baseline (n = 95). |
In the cross-fostering experiments, when two nests at the egg stage were cross-fostered, 67.39% of females (31 of 46; χ2 = 5.57, P = 0.018) returned to incubate foreign eggs. Whether females resumed their incubation behaviors was unrelated to the changed number of eggs or incubation days (Table 2), suggesting a consistently high probability for parents to accept unrelated offspring at the egg stage (Fig. 2D). When two nests at the chick stage were cross-fostered, 83.33% of parents (45 of 54 nests, χ2 = 24.00, P < 0.001) returned to the nest and provisioned foreign chicks. Whether parents resumed their provisioning behaviors was unrelated to nestling age but significantly related to the changed age between cross-fostered chicks (Table 2). The probability of parents provisioning unrelated young decreased with the age difference between fostered chicks (Fig. 2E).
Stage | Predictor variables | β ± SE | n | z | P |
Both nests at the egg stage | Intercept | −0.73 ± 0.31 | 46 | −2.31 | 0.02 |
Changed number of eggs | 0.05 ± 0.23 | 46 | 0.22 | 0.83 | |
Changed incubation days | 0.001 ± 0.05 | 46 | 0.02 | 0.98 | |
Both nests at the chick stage | Intercept | −9.87 ± 3.95 | 32 | −2.50 | 0.01 |
Age of the fostered nestlings | 0.31 ± 0.19 | 32 | 1.64 | 0.10 | |
Changed age of the fostered nestlings | 0.79 ± 0.38 | 32 | 2.06 | 0.04 |
When eggs in a nest were cross-fostered with chicks in another nest, incubation parents advanced their parenting behaviors from incubation to provisioning in 92.00% of nests (23 of 25 nests; χ2 = 17.64, P < 0.001), while provisioning parents reverted their parenting behaviors to incubation in 76.00% of nests (19 of 25 nests; χ2 = 6.76, P = 0.009). Whether incubation parents carried out the provisioning of foreign chicks was unrelated to incubation days of eggs, the number or changed number of offspring (Table 3). Whether provisioning parents carried out the incubation of foreign eggs was unrelated to the number or changed number of offspring, but significantly related to the age of their own chicks (Table 3). Parents had a higher possibility to advance than to revert their parenting behaviors in this cross-fostering experiment (Fig. 2F). The probability of provisioning parents accepting unrelated eggs decreased significantly with the age of their own chicks (Fig. 2F).
Predictor variables | Parameters of the model | |||||
At the egg stage (n = 25) | At the chick stage (n = 25) | |||||
β ± SE | z | P | β ± SE | z | P | |
Intercept | 0.14 ± 4.78 | 0.03 | 0.98 | −4.85 ± 4.77 | −1.02 | 0.31 |
Number of nestlings | −0.43 ± 0.72 | −0.59 | 0.55 | 0.17 ± 0.81 | 0.21 | 0.84 |
Changed number of nestlings | −1.10 ± 1.02 | −1.08 | 0.28 | −0.02 ± 0.74 | −0.03 | 0.98 |
Nestling age | 0.28 ± 0.21 | 1.33 | 0.19 | 0.46 ± 0.21 | 2.22 | 0.03 |
In the first nest duplication experiment, the familiar offspring were situated in the duplicated nest while the unfamiliar unrelated chicks were positioned in the original nest. The focus parents delivered food to the duplicated nest in 53 cases and to the original nest in 43 cases (χ2 = 1.04, df = 1, P = 0.307). Whether parents provisioned their own offspring was significantly related to the age but not number of their offspring (Table 4). The probability of parents provisioning unrelated young decreased significantly with the age of their offspring (Fig. 3C).
Fixed effects | β ± SE | n | z | P |
Intercept | −1.20 ± 1.16 | 96 | −1.04 | 0.30 |
Number of nestlings | −0.05 ± 0.24 | 96 | −0.22 | 0.82 |
Age of parents' offspring | 0.17 ± 0.08 | 96 | 2.08 | 0.04 |
In the second nest duplication experiment, the familiar cross-fostered chicks were situated in the duplicated nest while the unfamiliar offspring in the original nest. The focus parents delivered food to the duplicated nest in fifteen cases and only once to the original nest (Fig. 3D; χ2 = 12.25, df = 1, P < 0.001). Obviously, this result differed significantly from that in the first nest duplication experiment.
As offspring phenotypic traits would become individualized and fixed after a special developmental stage, parents could acquire the capacity for offspring recognition gradually by associating with them. The present study revealed that any factors that deprived parental association with their offspring or introduced unrelated young into the nest prior to this critical timeline would influence parental recognition of their offspring, hence leading to the occurrence of alternative parenting behaviors.
Individuals breeding in environments with food scarcity or higher risk of predation may exhibit an alternative parenting behavior during offspring provisioning (e.g., rejecting a partial of nestlings to ensure the survival of the remaining; Stoleson and Beissinger, 1997; Forbes et al., 2002; Zhu et al., 2023). These widespread phenomena indicate that rejecting their own offspring is actually a condition-dependent decision for parents, irrespective of whether they have the capacity for offspring recognition. For instance, parent birds are more likely to abandon current brood with the increasing risk of nest predation (Martin, 1995). In the present study, our nest-moving procedure in the nest resettlement experiment, i.e., the alteration of nest position (Fig. 1A and B), does look like a scene of nest predation. From the experimental results alone, there is a possibility that parental responses to risk can also result in the increased probability of rejecting their own offspring with nest-moving distances (Fig. 1C). However, as Azure-winged Magpies in our study area always experience high pressure of nest predation caused by predators or conspecific nest-raiders (Ren et al., 2016; Gao et al., 2022) and parents will not abandon the nest even if there is only one egg or chick left, it will be difficult for using parental responses to risk to explain why parents rejected their own offspring in a manipulated nest in the experiment. Alternatively, parental dependence on nest spatial position in offspring recognition is relatively a better explanation: along with the increase of nest-moving distance, the certainty that parents perceive a manipulated nest as their own will be lowered. Consequently, their probability of rejecting a manipulated nest increased (Fig. 1C).
It has been widely observed in animals that parental capacity for offspring recognition exhibits a temporal variation with offspring growth. For instance, parents in Bank Swallow (Riparia riparia) (Beecher et al., 1981), mammals (Jovanovic et al., 2000) and even social insects (Müller and Eggert, 1990), have reduced their dependence on nest spatial position in recognizing their own offspring, they instead rely largely on offspring phenotypic traits. In the present study, parental probability of rejecting their own offspring deceased with nestling age (Fig. 1D) provided new evidence for such a pattern in the Azure-winged Magpie. As offspring phenotypic traits become individualized and fixed with their growth, parents can acquire the capacity for offspring recognition by learning the developmental status of offspring phenotypic traits.
Parents accepting unrelated offspring may attribute to two reasons: one is that they are unable to recognize offspring, such as in cases of nest parasitism (Sorenson and Payne, 2002; Bouwman et al., 2005); the other is that parents are able to recognize offspring but recognizing and rejecting unrelated young is costly for them. For instances, when their own offspring and unrelated young with similar phenotypic traits are mixed together, the rejection of a suspected unrelated young increases the risk of rejecting their own offspring (Cherry and Bennett, 2001; Soler et al., 2003; Akkaynak and Stoddard, 2023). In our cross-fostering experiments conducted in Tibetan Azure-winged Magpies, we adopted two new ideas different from previous studies. Firstly, we cross-fostered the entire brood between two nests, which ensured that parents were exposed solely to unrelated chicks. Secondly, we established a gradient of age differences among cross-fostered offspring, even exchanging eggs with chicks, to ensure that parents could detect the change of offspring signals. If parents have the capacity for offspring recognition, they should reject the foreign eggs or chicks in the experiment. Our results showed that parents tended to incubate foreign eggs (Fig. 2D and E) and to provision foreign chicks at early chick stage (Fig. 2E and F) but did not exhibit the same patterns any more at later stage. These results further provided supports for an increased parental capacity for offspring recognition with the growth of offspring.
Regarding the second explanation for why parents accept unrelated young, namely that recognizing and rejecting unrelated offspring incurs significant costs for parents, in terms of the survival of their own offspring, our cross-fostering experiments do not support this hypothesis. By cross-fostering the entire brood between two nests, we ensured that parents were exclusively exposed to unrelated chicks. If parents were capable of recognizing their own offspring, they would have abandoned the nest due to the complete absence of their own offspring in a manipulated nest.
The cross-fostering procedure has been widely employed as a method for investigating the influence of parental effects on the development of offspring phenotypic traits and behaviors (Li and Zhang, 2010). Previous studies have indicated that the outcomes of cross-fostering experiments are closely associated with the initiation time of the experiment (Lefevre et al., 1998; Becciu et al., 2021), which can be attributed to parent−offspring association. Prior to establishing a strong parent−offspring association, parental effects on offspring development may exhibit greater strength, whereas after such an association is established, this effect may significantly diminish. In the Azure-winged Magpie, we designed cross-fostering experiments involving different-aged offspring, aiming to demonstrate how the time of depriving parent−offspring association affected parental recognition of foreign offspring. Results in both Fig. 2E−F and Fig. 3C indicate that when parents were deprived association with their offspring at early chick stage, they did not acquire the capacity for offspring recognition but remained depending on the nest spatial position in locating their offspring. As a result, their probability of accepting unrelated young maintained at higher level. By contrast, in those experiments carried out at later chick stage, parents may have acquired the capacity for offspring recognition through association with their offspring, resulting in the decrease of their probability accepting unrelated young.
The results of the second nest duplication experiment revealed a complete rejection of their own offspring by parents, while simultaneously accepting unrelated cross-fostered young (Fig. 3D), providing further support for the hypothesis that early parent−offspring association is crucial for parental capacity in recognizing offspring. Deprivation of parental association with their offspring during the early chick stage led to the establishment of recognition for cross-fostering unrelated young. The significant disparity between the outcomes of the first and second nest duplication experiments (Fig. 3D vs. 3C) emphasizes that parents establish their capacity for offspring recognition no later than day 11 under natural conditions. Integrating temporal variations from regression lines in Fig. 2E−F, it appears that parents may start learning offspring phenotypic traits as potential signals for recognition around day eight, but they may require an additional 2–3 days to full acquire the capacity for recognizing their offspring.
By investigating parental care behaviors in response to experimentally manipulated offspring signals in the Azure-winged Magpie, we demonstrated that parents can progressively acquire the capacity for offspring recognition with offspring growth via early association with their offspring. Any factors that disrupt parent−offspring association or introduce unrelated young into the nest before offspring phenotypic traits fully develop can impact parental capacity for offspring recognition, hence resulting in the occurrence of alternative parenting behaviors in provisioning the offspring.
Lifang Gao: Writing – original draft, Methodology, Formal analysis, Investigation, Writing – review & editing. Wen Zhang: Methodology, Investigation. Wenjing Zhu: Methodology, Investigation. Yichen Wu: Methodology, Investigation. Ran Xu: Methodology, Investigation. Ningning Sun: Methodology, Investigation, Conceptualization. Yujie Wang: Investigation, Methodology. Biyun Jia: Methodology, Investigation. Bo Du: Supervision, Methodology, Investigation, Funding acquisition, Resources, Writing – review & editing.
The ethical permits for life history data collection and individual sampling were issued by the Ethical Committee of School of Life Sciences, Lanzhou University (EAF2020002, EAF2021020). All works in the field were carried out under the Wildlife Conservation Law of the Thirteenth National People’s Congress of China (20170101, 20181026).
Datasets supporting the statistical analyses can be accessed from the Dryad Digital Repository, https://doi.org/10.5061/dryad.6q573n630.
The authors declare that they have no known competing financial interests or personal relationships that could have appeared to influence the work reported in this paper.
Authors will thank Qingmiao Ren, Xinwei Da, Guoliang Chen, Lili Xian, Juanjuan Luo, Haiyang Zhang, Fangyuan Luo, and Qian Wang for their assistance in the collection of life history data in the past eight years.
Boismenu, C., Gauthier, G., Larochelle, J., 1992. Physiology of prolonged fasting in greater snow geese (Chen caerulescens atlantica). Auk 109, 511–521.
|
Cherel, Y., Le Maho, Y., 1985. Five months of fasting in king penguin chicks: body mass loss and fuel metabolism. Am. J. Physiol. 249, R387–R392.
|
Daan, S., Masman, D., Groenewold, A., 1990. Avian basal metabolic rates: their association with body composition and energy expenditure in nature. Am. J. Physiol. 259, R333–R340.
|
Hohtola, E., 2012. Thermoregulatory adaptations to starvation in birds. In: McCue, M.D. (Ed.), Comparative Physiology of Fasting, Starvation, and Food Limitation. Springer-Verlag, San Antonio, TX, USA, pp. 157–170.
|
Hu, S.N., Zhu, Y.Y., Lin, L., Zheng, W.H., Liu, J.S., 2017. Temperature and photoperiod as environmental cues affect body mass and thermoregulation in Chinese bulbuls, Pycnonotus sinensis. J. Exp. Biol. 220, 844–855.
|
Liang, Q.J., Zhao, L., Wang, J.Q., Chen, Q., Zheng, W.H., Liu, J.S., 2015. Effect of food restriction on the energy metabolism of the Chinese bulbul (Pycnonotus sinensis). Zool. Res. 36, 79–87.
|
Liu, J.S., Li, M., 2006. Phenotypic flexibility of metabolic rate and organ masses among tree sparrows Passer montanus in seasonal acclimatization. Acta Zool. Sin. 52, 469–477.
|
MacKinnon, J., Phillipps, K., 2000. A Field Guide to the Birds of China. Oxford University Press, London, pp. 491–493..
|
McCue, M.D., 2010. Starvation physiology: reviewing the different strategies animals use to survive a common challenge. Comp. Biochem. Physiol. A 156, 1–18.
|
Pinheiro, E.C., Taddei, V.A., Migliorini, R.H., Kettelhut, I.C., 2006. Effect of fasting on carbohydrate metabolism in frugivorous bats (Artibeus lituratus and Artibeus jamaicensis). Comp. Biochem. Physiol. B 143, 279–284.
|
Schmidt-Nielsen, K., 1997. Animal Physiology: Adaptation and Environment. Cambridge University Press, Cambridge..
|
Swanson, D.L., 2010. Seasonal metabolic variation in birds: functional and mechanistic correlates. In: Thompson, C.F. (Ed.), Current Ornithology, vol. 17. Springer, Berlin, pp. 75–129.
|
Zhang, Y.F., Eyster, K., Liu, J.S., Swanson, D.L., 2015. Cross-training in birds: cold and exercise training produce similar changes in maximal metabolic output, muscle masses and myostatin expression in house sparrows (Passer domesticus). J. Exp. Biol. 218, 2190–2200.
|
Zhao, Z.J., Liu, Y.A., Xing, J.Y., Zhang, M.L., Ni, X.Y., Cao, J., 2014. The role of leptin in striped hamsters subjected to food restriction and refeeding. Zool. Res. 35, 262–271.
|
Zheng, W.H., Li, M., Liu, J.S., Shao, S.L., 2008b. Seasonal acclimatization of metabolism in Eurasian tree sparrows (Passer montanus). Comp. Biochem. Physiol. A 151, 519–525.
|
Zheng, W.H., Lin, L., Liu, J.S., Xu, X.J., Li, M., 2013. Geographic variation in basal thermogenesis in little buntings: relationship to cellular thermogenesis and thyroid hormone concentrations. Comp. Biochem. Physiol. A 164, 240–246.
|
Predictor variables | β ± SE | n | z | P | |||||||||||||||
Intercept | 3.30 ± 1.75 | 179 | 1.88 | 0.06 | |||||||||||||||
Offspring age (day) | 0.34 ± 0.08 | 179 | 4.36 | < 0.001 | |||||||||||||||
Nest-moving distance (m) | −2.44 ± 0.39 | 179 | −6.22 | < 0.001 | |||||||||||||||
At the chick stagea | 4.91 ± 0.82 | 84 | 6.01 | < 0.001 | |||||||||||||||
aNote: the value at the egg stage was set as the baseline (n = 95). |
Stage | Predictor variables | β ± SE | n | z | P |
Both nests at the egg stage | Intercept | −0.73 ± 0.31 | 46 | −2.31 | 0.02 |
Changed number of eggs | 0.05 ± 0.23 | 46 | 0.22 | 0.83 | |
Changed incubation days | 0.001 ± 0.05 | 46 | 0.02 | 0.98 | |
Both nests at the chick stage | Intercept | −9.87 ± 3.95 | 32 | −2.50 | 0.01 |
Age of the fostered nestlings | 0.31 ± 0.19 | 32 | 1.64 | 0.10 | |
Changed age of the fostered nestlings | 0.79 ± 0.38 | 32 | 2.06 | 0.04 |
Predictor variables | Parameters of the model | |||||
At the egg stage (n = 25) | At the chick stage (n = 25) | |||||
β ± SE | z | P | β ± SE | z | P | |
Intercept | 0.14 ± 4.78 | 0.03 | 0.98 | −4.85 ± 4.77 | −1.02 | 0.31 |
Number of nestlings | −0.43 ± 0.72 | −0.59 | 0.55 | 0.17 ± 0.81 | 0.21 | 0.84 |
Changed number of nestlings | −1.10 ± 1.02 | −1.08 | 0.28 | −0.02 ± 0.74 | −0.03 | 0.98 |
Nestling age | 0.28 ± 0.21 | 1.33 | 0.19 | 0.46 ± 0.21 | 2.22 | 0.03 |
Fixed effects | β ± SE | n | z | P |
Intercept | −1.20 ± 1.16 | 96 | −1.04 | 0.30 |
Number of nestlings | −0.05 ± 0.24 | 96 | −0.22 | 0.82 |
Age of parents' offspring | 0.17 ± 0.08 | 96 | 2.08 | 0.04 |