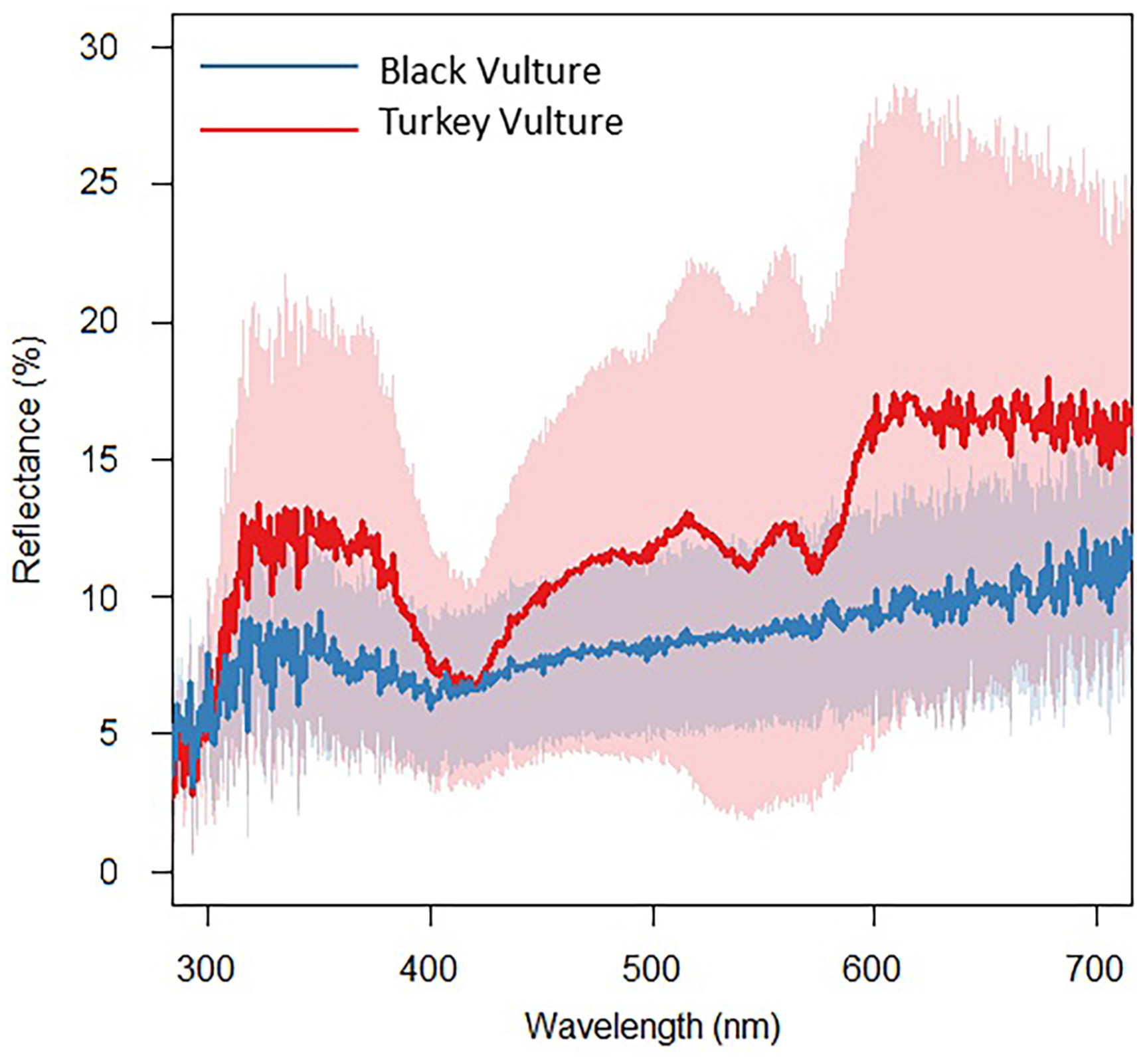
Citation: | Nicholas M. Justyn, Matthew J. Powers, Geoffrey E. Hill, Kayla Alexander, Adrián Naveda-Rodríguez, Scott A. Rush. 2023: The mechanisms of color production in black skin versus red skin on the heads of New World vultures. Avian Research, 14(1): 100071. DOI: 10.1016/j.avrs.2022.100071 |
A determination of how the color of animal integument is produced is a starting point for investigations into the function and evolution of coloration. The mechanisms that give rise to the color of bare skin of New World vultures are largely unexplored. Here, we investigate the source of color production in the bare skin of Turkey Vultures (Cathartes aura) and Black Vultures (Coragyps atratus). Using UV–vis reflectance spectroscopy, we found evidence that hemoglobin is the primary pigment responsible for the red coloration of the bare skin on the heads of Turkey Vultures, and that eumelanin is responsible for the black coloration of the bare skin on the heads of Black Vultures. Light microscopy of incisional skin samples further supported these mechanisms of color production by revealing the presence of numerous blood vessels near the surface of the Turkey Vulture skin, and a high concentration of melanosomes in the skin of Black Vultures. Using high performance liquid chromatography (HPLC), we detected carotenoids within the skin of both species with significantly higher total concentrations of carotenoids in the skin of Turkey Vultures compared to the skin of Black Vultures. The carotenoids detected were dietary carotenoids that typically produce yellow coloration when accumulated in integument and were present in low concentrations. We hypothesize that the dietary carotenoids present do not contribute to the color of the skin, but rather help to compensate for the lack of melanosomes found in Turkey Vulture skin. The presence of additional carotenoids may act as an antioxidant to minimize UV damage when the bare Turkey Vulture head skin is exposed to direct sunlight for prolonged periods of time when soaring and scavenging for food.
The mechanisms that produce feather coloration have received substantial research attention, but far fewer studies have examined the mechanisms that give rise to the coloration of bare skin in birds (Hill and McGraw, 2006; Davis and Clarke, 2022). The color production mechanisms promoting the color of the bare heads of carrion-eating birds is a prime example of the limits of current knowledge regarding skin coloration. In both Old World vultures (Order Accipitriformes) and New World vultures (Order Cathartiformes), many species lack feathers on their heads and necks as an adaptation to feeding on carcasses and for thermoregulation (Ward et al., 2008). The bare heads of these birds are most commonly black, red, orange, or yellow (del Hoyo et al., 1992). A common assumption is that black head coloration results from eumelanin deposited in the skin, but the source of red coloration in the heads of many vultures is unknown (Nicolaï et al., 2020). With multiple potential mechanism for the production of skin coloration such as red or black, visual assessment is not adequate for determining how a particular visual display is achieved — analysis of pigments and nanostructures is required (McGraw et al., 2004). Even mechanisms that produce similar colors can be the result of completely different biological processes and evolutionary forces (Iverson and Karubian, 2017; Orteu and Jiggins, 2020). For example, while ketolated carotenoids are the most common source of red coloration in bird integument, birds can also achieve red skin coloration by using hemoglobin as the primary chromatophore (Toral et al., 2008). By passing highly oxygenated blood near the surface of the skin, birds can flush and turn their skin red, sometimes in combination with carotenoids (Negro et al., 2006).
Here, we examined the skin and blood of two New World vultures, the Turkey Vulture (Cathartes aura) and the Black Vulture (Coragyps atratus) to: 1) determine the mechanisms that produce the red head coloration of mature adults of the former and the black head coloration of the latter; and 2) hypothesize the functional consequences for these differences. A prior study of the yellow skin on the heads of Egyptian Vultures confirmed that vultures are capable of using carotenoids to color their skin (Negro et al., 2002). However, the mechanisms used by other vultures to create their skin color are unknown. We quantified the color of the skin using UV–vis reflectance spectroscopy, examined the structure of the skin using light microscopy, and quantified the concentration of carotenoids present in the skin and blood of each species.
All work involving live birds for this study was carried out under federal (USGS #23835) and state (Mississippi permit for SAR) permits, as well as an IACUC protocol (18–551). Black Vultures (n = 7) and Turkey Vultures (n = 7) were captured using baited walk-in traps (Wildlife Dominion Management LLC) set at two locations in Mississippi (33.5256° N, 88.6721° W; 32.3757° N, 88.6133° W). Trap sites are within solid waste management facilities and are separated by 130 km. Traps were baited with deer collected from local roadways after vehicular-related mortalities.
Trapping was carried out on November 22–23, 2021. Birds were captured and transferred to a holding container prior to processing. The age of each Turkey Vulture (HY = Hatching Year (birds < 1 year old); SY = Second Year; ASY = After Second Year) was determined by a combination of head and beak color as described by Henckel (1981). Black Vultures were aged by the amount of feathering on their head, as well as beak color (see Pyle, 2008). Approximately 2 mL of blood was sampled from the right basilic vein. Blood was collected from the basilic vein using a 25 g, 1.6 cm needle affixed to a 2 mL syringe (BD Integra) and transferred to a 3 mL non-heparinized phlebotomy tube. A small sample of blood was transferred to blotting paper for use in assigning the sex to each bird genetically, an assessment carried out by Animal Genetics (Tallahassee, Florida).
We applied a small (~1/2 cm strip) of capsaicin cream (0.1% Capsaicin; Capzasin HP) to the skin of the nape of each bird, a location distal to the parietal bone, approximately ~2 cm anterior to the top of the bird's eye. After the cream was allowed to absorb into the skin, a time of approximately 2 min, sterile forceps were used to grasp the skin, gently pulling back posterior to the head. Sterile, surgical scissors were then used to excise a small 1–2 cm2 of skin that included the epidermis and dermis. Once excised, this skin sample was transferred to a 20 mL, sterile, glass scintillation vial. Styptic powder (Dogswell; Whitebridge Pet Brands) was then spread into the incision on the bird and the area treated with a thin covering of NewSkin (0.2% Benzethonium chloride; Advantice Health LLC). The bird was then held for 2 min to ensure that the bleeding had been staunched and there was no obvious changes in the bird's behavior. If the bird did not show abnormal behavior and there was no bleeding noted from the wound, each bird was released.
Blood and skin samples were immediately transferred to a cooler and stored on ice until additional processing away from the field site. Upon returning to the lab (Mississippi State University), containers of blood and skin biopsies were purged using gaseous nitrogen then stored in a −80 ℃ freezer until shipment to Auburn University.
We used UV–vis reflectance spectroscopy to quantify the color of Turkey (n = 5) and Black Vulture (n = 5) skin samples, prior to carotenoid extraction. Each skin sample was placed on a black cardboard background, ventral side up, and gently pressed flat with tweezers. We obtained three measurements from each skin sample at a 90° angle of incidence in a dark room. We used an Ocean Optics USB4000 spectrophotometer connected to an Ocean Optics PX-2 pulsed xenon light source to obtain all of the measurements reported. Prior to capturing reflectance spectra, we calibrated our measurements using a white reflectance standard (Labsphere Inc., North Sutton, NH, USA). We captured multiple measurements from each skin sample to account for any variation in color within samples. The spectral data obtained were acquired using OceanView 1.67, and then analyzed and visualized using the pavo package in R (Maia et al., 2013, 2019; R Core Team, 2020).
We took a single representative incisional skin sample from a Turkey (n = 1) and Black Vulture (n = 1) and placed them in 10% neutral buffered formalin for at least 48 h prior to processing at the College of Veterinary Medicine histology laboratory of Mississippi State University. Samples were either bisected longitudinally or placed whole into a tissue cassette. Each section was routinely processed, paraffin embedded, sectioned at 5 μm, and stained with hematoxylin and eosin (H & E) for light microscopy.
We performed carotenoid extractions from 100 μL of Turkey (n = 5) and Black Vulture (n = 5) plasma in 500 μL acetone. We first added acetone to the plasma, vortexed for 10 s, and then sonicated at 10 W for 10 s. We used centrifugation to pellet and remove cellular debris, saving the carotenoid extract suspended in acetone. The carotenoid extract from the blood plasma was evaporated to dryness, resuspended in 60 μL acetone, and capped with nitrogen for immediate HPLC analysis. Prior to extracting carotenoids from the Turkey (n = 5) and Black Vulture (n = 6) skin, we cut a small segment of skin and thoroughly washed it with water and ethanol. We then dried and weighed the sample to standardize the extracted carotenoid concentrations (conc) by tissue mass. The final mass of the skin tissue in grams used from each bird can be found in the supplemental data file, but summary statistics are reported here for convenience (all values in grams: average mass = 0.046; median mass = 0.043; min = 0.031; max = 0.073; st.dev = 0.014). Skin samples were homogenized in 500 μL acetone first by grinding with a pestle and mortar and then by sonication at 10 W for 10 s. While uncommon in plasma carotenoids, some avian species add fatty acid esters to carotenoids in their soft tissue like skin. To remove potential fatty esters from the skin carotenoids, we performed a saponification (Toomey and McGraw, 2007). We dried our carotenoid extract and resuspended in 250 μL ethanol. We then added 100 μL 0.02 M KOH dissolved in methanol and vortexed for 10 s. We then added 250 μL water, 500 μL methyl-tert-butyl-ether, and 250 μL hexane and vortexed for 30 s. Finally, we added 100 μL of saturated saltwater and shook the tubes vigorously for 1 min to transfer the carotenoids to the organic layer. This process was repeated twice to minimize carotenoid loss. The organic phase containing carotenoids was transferred to a new tube, dried down, resuspended in 70 μL acetone, and capped with nitrogen for immediate HPLC analysis.
We separated and quantified carotenoids using HPLC following the methods of Weaver et al. (2018a) and Wright et al. (1991). We injected 10 μL of carotenoid extract in acetone on to a Sonoma C18 column (10 μm, 250 × 4.6 mm, ES Technologies, New Jersey, USA) fitted with a C18 guard cartridge. Carotenoids were separated using a Shimadzu Prominence HPLC system with mobile phases A 80:20 methanol: 0.5 M ammonium acetate, B 90:10 acetonitrile: water, and C ethyl acetate in a tertiary gradient of 100% A to 100% B over 4 min, then to 80% C: 20% B over 14 min, back to 100% B over 3 min, and returning to 100% A over 5 min and held for 6 min (Wright et al., 1991; Weaver et al., 2018a). We visualized and detected carotenoid absorbance using a Prominence UV/Vis detector set to a wavelength of 450 nm. We identified and quantified carotenoids by comparison to authentic standards that included astaxanthin, zeaxanthin, β-carotene, lutein, 3-hydroxyechinenone, and canthaxanthin. We normalized carotenoid concentration by the dry weight or plasma volume of each skin and blood sample, respectively (reported as μg carotenoid per g tissue). For carotenoids in the tissue samples for which we had no pure standard for quantification (echinenone, α-cryptoxanthin, β-cryptoxanthin), we used the quantification curve for 3-hydroxyechinenone to calculate their mass standardized concentrations, as is required practice when lacking a matching pure standard (Blanco et al., 2013; Richins et al., 2014).
All statistical analyses were performed in R (R Core Team, 2020) using the following packages: 'lme4' (Bates et al., 2014), 'Hmisc' (Harrell Jr and Dupont, 2021), 'lmerTest' (Kuznetsova et al., 2017), 'agricolae' (de Mendiburu, 2014), 'MASS' (Venables and Ripley, 2013), 'emmeans' (Lenth et al., 2018), 'car' (Fox and Weisberg, 2018), 'sf' (Pebesma, 2018), 'rstatix' (Kassambara, 2021), and 'MuMIn' (Barton, 2009). Data wrangling was performed in R with the help of the follow packages: 'tidyverse' (Wickham, 2017), 'dplyr' (Wickham et al., 2015), 'reshape2' (Wickham, 2007), 'plotrix' (Lemon, 2006), and 'HH' (Heiberger and Burt Holland, 2015). Figures were produced using the following packages: 'ggpubr' (Kassambara, 2018), 'cowplot' (Wilke, 2016), 'RColorBrewer' (Neuwirth, 2014), and 'ggjoy' (Wilke, 2017). For a full review of our statistical analyses, please refer to the annotated R-code included in the supplemental material; we provide below a brief description of each analysis and the type of modeling used.
We normalized all carotenoid concentration data by the mass of tissue from which we extracted the pigment. We transformed carotenoid concentrations to fit model assumptions of normality using a log10 transformation. To examine the overall effects on carotenoid concentrations among individuals in our dataset, we first performed a two-way ANOVA on the following categorical variables and their pairwise interactions: sex (male vs. female), age (after second year [ASY] vs. second year [SY] or younger), species (Black vs. Turkey Vulture), and tissue type (skin vs. blood). Only one individual bird was a SY bird (ID#: R59). So, we combined data from that bird with the hatch year individuals to make the two broad age groupings ASY and SY. All other birds besides R59 in the SY group were hatch year individuals. We also tested for pairwise interactions between each of these four variables. We performed this analysis for total carotenoid concentrations, zeaxanthin concentration, β-carotene concentration, lutein concentration, and the concentration of the "minor" carotenoids detected in low abundance (echinenone, α-cryptoxanthin, and β-cryptoxanthin). Due to a repeatedly observed interaction between tissue and species (see results below), we blocked data by tissue and species together for linear modeling.
To examine the differences in carotenoid concentrations between Black Vultures and Turkey Vultures, we fit linear mixed effects model with individual age group as a random effect to account for potential non-independence of data obtained from birds of the same age group. As noted above, tissue type and species were blocked as a single variable in the analysis because of a significant interaction between the two separate variables. Pairwise differences between each tissue and species were estimated from this model using the 'emmeans' package, after applying a Tukey's correction for Type 1 error due to repeated pairwise comparisons.
To examine differences in carotenoid concentrations between after second year (ASY) and hatch/second year birds (SY), we fit a linear mixed model with tissue type as a random effect to control for non-independence of carotenoid concentration data from skin and blood within each age group. The age and species group variables were blocked together, to account for any potential interactions between the two variables (we detected this effect with minor carotenoid concentrations). However, pairwise differences between each age group and species were estimated from this model also using 'emmeans'.
We analyzed differences due to sex using a linear mixed model in a similar manner as described above. Even though we detected no interaction between species and sex, to control for the overwhelming effect of species on the data, we blocked the variables by species and sex. We included a random effect of tissue type, as we did with the linear model fit to examine differences between the age groups. Pairwise differences between each sex and species were estimated from this linear model using 'emmeans'. All statistical tests were deemed to be significant at α = 0.05.
We found that the reflectance spectra obtained from the Turkey Vulture skin samples (Fig. 1) did not match any of the spectra for other pigments commonly deposited within red colored integument (Toral et al., 2008). The high amount of reflectance in the middle of the spectrum from 450 to 550 nm is not characteristic of carotenoids, which typically absorb these wavelengths of light, even if combined with structures (Justyn et al., 2022a, b). Instead, the reflectance spectra closely resemble that of hemoglobin (Negro et al., 2006; Bender et al., 2009; Kim et al., 2014). The reflectance spectra of the Black Vulture skin (Fig. 1) closely resemble that of eumelanin (Toral et al., 2008). While all skin samples visibly maintained coloration when sampled, it is possible that the skin samples may have faded since the time of collection. This could have resulted in less bright reflectance spectra when compared to reflectance spectra obtained directly from living vulture skin.
We found very few melanosomes scattered across the skin sample of the Turkey Vulture (Fig. 2A), and a much denser presence of melanosomes in the Black Vulture skin sample (Fig. 2B). There was also an absence of lipid filled vacuoles present in either sample, suggesting that carotenoids are not deposited in significant concentrations in the skin of either species. When observing the histological structures present in the skin samples, there are numerous blood vessels near the surface of the Turkey Vulture skin. The layer of collagen in the Turkey Vultures appears thicker than the layer in the Black Vultures, although both are likely too thin to produce structural color (Prum and Torres, 2003).
We detected six primary carotenoids in the skin and blood of Turkey and Black Vultures (Appendix Fig. S1). These included lutein, zeaxanthin, α-cryptoxanthin, β-cryptoxanthin, echinenone, and β-carotene (Appendix Fig. S1). These carotenoids were detectable in all samples from both species (Appendix Fig. S2). We observed that the three most abundant carotenoids across all samples were lutein, zeaxanthin, and β-carotene (Fig. 3). The other three carotenoids detected, α-cryptoxanthin, β-cryptoxanthin, echinenone were present in much lower concentrations — we refer to these three as "minor carotenoids" in these species here forward. Across the two species, the skin and blood, the two sexes, and the two age groups analyzed, we observed similar proportions of these carotenoids (Fig. 3).
We observed a statistically significant effect of species on the carotenoid concentrations measured across all samples (Species – dfn = 1, dfd = 7, F = 7.11, p = 0.0322). We also observed a marginal interaction between species and tissue type (Species: Tissue – dfn = 1, dfd = 7, F = 4.01, p = 0.0854). There was a significant effect of species and marginal effect of age on zeaxanthin concentration (Species – dfn = 1, dfd = 6, F = 14.45, p = 0.0090; Age – dfn = 1, dfd = 6, F = 4.53, p = 0.0774). For the concentration of β-carotene across our sample data, we detected a marginal interaction between tissue type and species (Species: Tissue – dfn = 1, dfd = 7, F = 3.95, p = 0.0873). We detected no clear effect of any of our variables on lutein concentrations across the sample data. However, we did detect a significant effect of tissue type and species on the concentrations of the minor carotenoids echinenone, α-cryptoxanthin, and β-cryptoxanthin (Species – dfn = 1, dfd = 7, F = 12.16, p = 0.0102; Tissue type – dfn = 1, dfd = 7, F = 7.24, p = 0.0311). For the minor carotenoids, we observed a significant interaction between species and tissue type (Species: Tissue – dfn = 1, dfd = 7, F = 11.17, p = 0.0124).
When tissue concentrations were evaluated between species, we found that Turkey Vultures (Mean concentration in μg/g ± SE: 0.62 ± 0.12; values presented the same way hereinafter) accumulated significantly more carotenoids than Black Vultures (0.19 ± 0.11 μg/g; β = 1.40; t = 3.01 p = 0.0079). We found that Turkey Vulture skin (0.89 ± 0.15 μg/g) had, on average, significantly greater concentrations of carotenoids than Black Vulture skin (0.10 ± 0.14 μg/g; β = 2.30; t = −4.06; p = 0.0045; Fig. 4). This appeared to be driven by zeaxanthin, β-carotene, and minor carotenoid concentrations (Fig. 4). While not significantly different, Black Vulture blood was observed to hold higher concentrations of carotenoids (0.33 ± 0.16 μg/g) than Black Vulture skin (0.10 ± 0.14 μg/g; β = 1.10; t = 1.90; p = 0.2649), on average (Fig. 4).
We found that, on average, ASY individuals (0.54 ± 0.10 μg/g) sequestered higher concentrations of carotenoids in their tissues compared to SY (0.27 ± 0.13 μg/g). Although, this difference was not statistically significant (β = 0.58; t = −1.17; p = 0.2603). However, when making pairwise comparisons between the two species and age groups, we found only marginal statistical differences in a single comparison. We found that ASY Turkey Vultures (0.74 ± 0.14 μg/g) had, on average, higher concentrations of carotenoids than ASY Black Vultures (0.28 ± 0.12 μg/g; β = 1.47; t = −2.57; p = 0.0862; Appendix Fig. S3). This was broadly visible across the separate carotenoid concentrations as well (Appendix Fig. S3). However, we only observed a statistically significant difference between the zeaxanthin concentrations of ASY Turkey Vultures (0.21 ± 0.02 μg/g) and younger Black Vultures (0.02 ± 0.04 μg/g; β = 2.26; t = 4.01; p = 0.0055) or ASY Black Vultures (0.06 ± 0.03 μg/g; β = 1.53; t = −4.02; p = 0.0061; Appendix Fig. S3). For minor carotenoids, ASY Turkey Vultures (0.06 ± 0.02 μg/g) did have marginally greater concentrations than ASY Black Vultures (0.02 ± 0.02 μg/g; β = 0.96; t = −2.52; p = 0.0946; Appendix Fig. S3).
We found that the two sexes had similar concentrations of carotenoids in their tissues, with no significant differences detected between male (0.45 ± 0.13 μg/g) and female birds (0.36 ± 0.10 μg/g; β = 0.33; t = −0.67; p = 0.5107; Appendix Fig. S4). This same pattern was observed when looking at the specific carotenoid concentrations as well (Appendix Fig. S4).
The mechanisms that produce the various colors of bare skin that are displayed by different species of birds are poorly understood. Here, we analyzed blood as well as skin collected from the bare heads of two differently colored New World vulture species, the Turkey Vulture and Black Vulture, to characterize the mechanisms of color production and physiological differences between the two species. Using UV–vis reflectance spectroscopy and light microscopy, we found evidence that hemoglobin is the primary pigment responsible for the red coloration of the Turkey Vulture head skin, while eumelanin is responsible for the black coloration of the Black Vulture head skin.
To characterize the color production mechanisms present in the skin of both vulture species, we first performed UV–vis spectroscopy to examine the spectral reflectance patterns. UV–vis spectroscopy initially indicated that hemoglobin is primarily responsible for the red coloration of the Turkey Vulture skin and that eumelanin is responsible for the black coloration of the Black Vulture skin (Fig. 1). High reflectance between 450 and 550 nm indicated that red ketolated carotenoids, like astaxanthin, were not present in sufficient quantities to impact the skin color because ketolated carotenoids absorb light heavily within this range (Ilagan et al., 2005; Elde et al., 2012). We also observed that the skin from both species had a moderate levels of UV refection, with the Turkey Vulture skin being the most UV reflective. The moderate UV peak present is not typical for integument that contains melanin, which highly absorbs UV light. This could perhaps indicate that the structural organization of the collagen is slightly reflecting UV light in both species (Prum and Torres, 2003; Jourdie et al., 2004).
In an effort to search for possible structural differences between the two species, we performed light microscope histology. We observed a high number of melanosomes within the Black Vulture compared to the skin of the Turkey Vulture, further indicating that the black skin color is due to the presence of eumelanin (Fig. 2). We did not observe any lipid vacuoles containing carotenoids deposited in either of the skin samples, but we did observe numerous blood vessels near the surface of the Turkey Vulture's skin. The presence of an abundance of blood vessels further suggests that hemoglobin is the primary source of coloration for the red skin, and that Turkey Vultures also likely possess the ability for facial flushing (Negro et al., 2006).
In comparison to the Black Vulture skin sample, the Turkey Vulture skin also has a thicker layer of collagen, which could result in the increased UV reflection observed. Alternatively, this layer of collagen might play no role in the head coloration of Turkey Vultures and the increased UV reflectance could be due simply to the increased concentrations of hemoglobin near the surface of the skin (Prum and Torres, 2003; Bender et al., 2009; Kim et al., 2014). Additionally, vultures are thought to be sensitive to and capable of perceiving UV light (Ödeen and Håstad, 2013). The significant amount of UV reflectance seen in the Turkey Vulture skin would be visible by other vultures, and could play a role in signaling, regardless of the method of production.
We conclude that the red coloration of the bare heads of Turkey Vultures is a product of hemoglobin, but we did observe detectable levels of dietary carotenoids in the skin and blood from both species (Fig. 3). The detected carotenoid profiles we observed (lutein, zeaxanthin, α-cryptoxanthin, β-cryptoxanthin, echinenone, and β-carotene) matched those previously reported for the dark heads of Black Vultures and Andean Condors (Vultur gryphus; Blanco et al., 2013). Blanco et al. (2013) observed these same carotenoids in similar proportions in the plasma of Black Vultures, but found much higher levels of lutein relative to β-carotene in the Andean Condor, citing dietary differences due to increased vegetal consumption in the condors as the probable explanation for the disparity between those two species. We found significantly higher concentrations of lutein and β-carotene in the skin of Turkey Vulture compared to the skin of the Black Vulture (Fig. 4). Moreover, we observed that the skin of Turkey Vultures contained significantly more total carotenoids than the skin of Black Vultures. The difference in carotenoid concentrations between skin samples could be the result of more blood being trapped in the Turkey Vulture skin because of the abundance of blood vessels in this tissue, irrespective of the similar concentration of carotenoids in the blood (standardized by plasma volume) in both species (Fig. 4). We are confident, however, that the concentrations of yellow dietary carotenoids in the skin of Turkey Vultures were too low to significantly contribute to head coloration. Indeed, the concentrations detected were much lower than typically seen in the integument of other species confirmed to utilize carotenoids as the primary source of visible coloration (Negro et al., 2002; McGraw and Toomey, 2010).
If carotenoids are not contributing to coloration, why does the red-headed vulture species have higher concentrations of skin carotenoids compared with the black-headed species? Higher concentration of carotenoids in the heads of Turkey Vultures compared to the Black Vultures may help to compensate for the lack of melanin in their skin and serve as an antioxidant to minimize UV damage that Turkey Vultures receive while soaring in direct sunlight for long periods of time (Darvin et al., 2011; Lademann et al., 2011; Zerres and Stahl, 2020). Increased levels of carotenoids may not be necessary in the skin of Black Vultures, since the high levels of melanin present already protect skin from UV radiation (Nicolaï et al., 2020). Although, a meta-analysis of songbird studies and an experimental study of canaries presented evidence that carotenoids do not serve as important antioxidants in birds (Koch et al., 2018; Weaver et al., 2018b), these studies focused on fully feathered bird species that were protected from UV radiation by their feathers. Vultures are exposed to full sunlight for hours per day and the production of free radicals may be substantially higher in the exposed skin of vultures compared with the feathered bodies of songbirds. An increased concentration of carotenoids in the Turkey Vulture skin, combined with the UV reflection observed, may help the Turkey Vulture reduce the negative effects of high levels of UV radiation.
NMJ, MJP, GEH, AN, and SAR conceived the study. NMJ, MJP, and KA contributed to data collection and analysis. NMJ wrote the first draft. All authors contributed to the interpretation of results and writing of the final manuscript. All authors read and approved the final manuscript.
The authors confirm that all work involving live birds for this study was conducted under the ethical guidelines and protocols of federal (USGS #23835) and state (Mississippi permit for SAR) permits, as well as IACUC protocol (18–551).
The authors declare that they have no known competing financial interests or personal relationships that could have appeared to influence the work reported in this paper.
We would like to thank Dr. Paul Cobine at Auburn University for access to his HPLC. This work was provided for by the National Institute of Food and Agriculture, U.S. Department of Agriculture, McIntire-Stennis project under accession number MISZ-082100 and the National Science Foundation grant number IOS-2037741. This study was prepared under contract with Mississippi State University, PI Scott Rush, with financial support from the Office of Local Defense Community Adjustment, Department of Defense. The content reflects the views of the Mississippi State University, PI Scott Rush, and does not necessarily reflect the views of the Office of Local Defense Community Adjustment.
Supplementary data to this article can be found online at https://doi.org/10.1016/j.avrs.2022.100071.
Bates, D., Mächler, M., Bolker, B., Walker, S., 2014. Fitting Linear Mixed-Effects Models Using Lme4 arXiv preprint arXiv: 1406.5823.
|
de Mendiburu, F., 2014. Agricolae: Statistical Procedures for Agricultural Research. R package version 1.
|
del Hoyo, J., Elliott, A., Sargatal, J., 1992. Handbook of the Birds of the World, vol. 2. Lynx edicions, Barcelona.
|
Fox, J., Weisberg, S., 2018. An R Companion to Applied Regression. Sage Publications, Thousand Oaks.
|
Harrell Jr., F.E., Dupont, C., 2021. Hmisc: Harrell Miscelleneous. R Package Version.
|
Heiberger, R.M., Burt Holland, B.H., 2015. Statistical Analysis and Data Display: An Intermediate Course with Examples in R. Springer, New York.
|
Henckel, R.E., 1981. Ageing the Turkey vulture HY to ASY. N. Am. Bird Bander 6, 106–107.
|
Hill, G.E., McGraw, K.J., 2006. Bird Coloration: Mechanisms and Measurements, vol. 1. Harvard University Press, Cambridge.
|
Kassambara, A., 2018. Ggpubr: "Ggplot2" Based Publication Ready Plots. R package version 0.1.7.
|
Kuznetsova, A., Brockhoff, P.B., Christensen, R.H., 2017. lmerTest package: tests in linear mixed effects models. J. Stat. Software 82, 1–26.
|
Lemon, J., 2006. Plotrix: a package in the red light district of R. R. News 6, 8–12.
|
Lenth, R., Singmann, H., Love, J., Buerkner, P., Herve, M., 2018. Emmeans: estimated marginal means, aka least-squares means. R package version, 1(1), 3.
|
Maia, R., Eliason, C.M., Bitton, P.P., Doucet, S.M., Shawkey, M.D., 2013. pavo: an R package for the analysis, visualization and organization of spectral data. Methods Ecol. Evol. 4, 906–913.
|
Neuwirth, E., 2014. RColorBrewer: ColorBrewer Palettes. R Package Version 1, pp. 1–2.
|
Pyle, P., 2008. Identification Guide to North American Birds. Part Ⅱ: Anatidae to Alcidae. Slate Creek Press, Point Reyes Station.
|
Richins, R.D., Kilcrease, J., Rodgriguez-Uribe, L., O'connell, M.A., 2014. Carotenoid extraction and quantification from Capsicum annuum. Bio-Protocol 4, e1256.
|
Venables, W.N., Ripley, B.D., 2013. Modern Applied Statistics with S-PLUS. Springer Science and Business Media, New York.
|
Wickham, H., 2007. Reshaping data with the reshape package. J. Stat. Software 21, 1–20.
|
Wickham, H., 2017. Tidyverse: Easily Install and Load the 'tidyverse'. R Package Version 1.
|
Wickham, H., Francois, R., Henry, L., Müller, K., 2015. Dplyr: a Grammar of Data Manipulation. R package version 0.4.3.
|
Wilke, C.O., 2016. Cowplot: Streamlined Plot Theme and Plot Annotations for 'ggplot2. CRAN Repos 2 R2.
|
Wilke, C.O., 2017. Ggjoy: Joyplots in 'ggplot2'. R package version 0.3.0.
|
Zerres, S., Stahl, W., 2020. Carotenoids in human skin. BBA-Mol. Cell Biol. L. 1865, 158588.
|