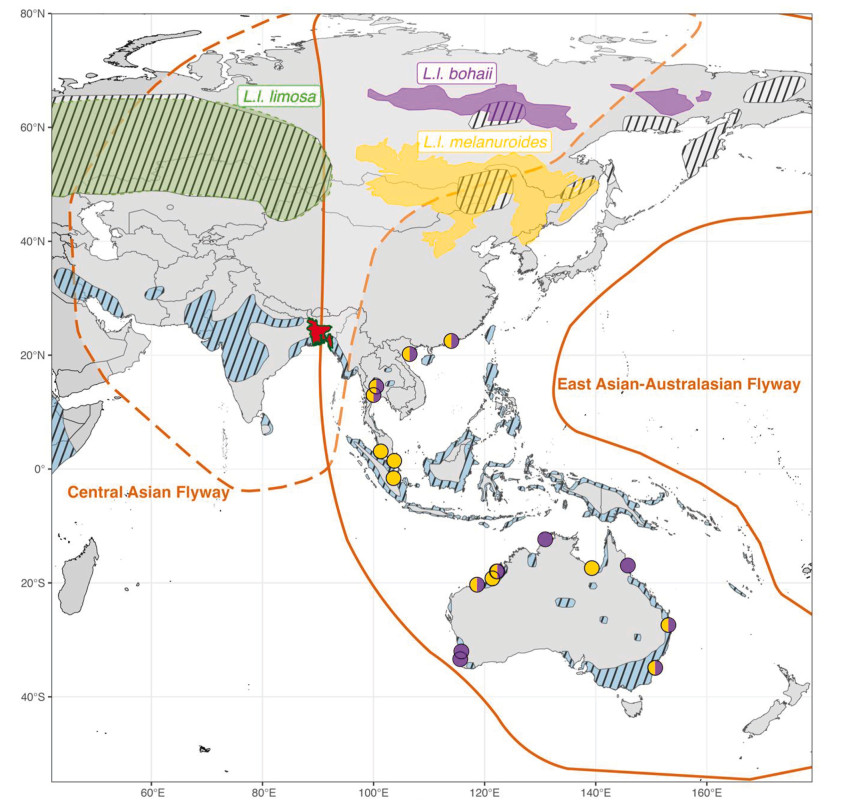
Citation: | Delip K. Das, Wouter M.G. Vansteelant, Bingrun Zhu, Shariful Islam, Naim Khandakar, Marco van der Velde, Chris J. Hassell, Jesse R. Conklin, Pierrick Bocher, Jos C.E.W. Hooijmeijer, Yvonne I. Verkuil, Theunis Piersma. 2025: Three subspecies of Black-tailed Godwit share non-breeding sites in the world’s largest river delta. Avian Research, 16(1): 100226. DOI: 10.1016/j.avrs.2025.100226 |
During the non-breeding season (September–April), Black-tailed Godwits (Limosa limosa) are commonly seen in coastal and inland wetlands of the Ganges-Brahmaputra-Meghna Delta in Bangladesh. We hypothesize that the Ganges-Brahmaputra-Meghna Delta, at the overlap between the Central Asian and East Asian–Australasian flyways, may host three subspecies that breed in disjunct areas of temperate and northern Asia: L. l. limosa, L. l. melanuroides, and L. l. bohaii. We used mitochondrial DNA (mtDNA) haplotype network and biometric analysis to determine subspecies in captured individuals, and deployed GPS–GSM transmitters to verify breeding areas of individuals with subspecies assignments. To test for differential habitat preferences, we sampled birds at two ecologically distinct habitats known to host the largest concentrations of non-breeding Black-tailed Godwits in Bangladesh: Nijhum Dweep National Park, a tidal coastal habitat with brackish water on the south-central coast, and Tanguar Haor (‘backmarsh’), a seasonal freshwater floodplain in the north. During the non-breeding seasons of 2021–2022. and 2022–2023., we sampled and measured 93 Black-tailed Godwits, 54 of which were equipped with GPS–GSM transmitters. Our mtDNA haplotype network analysis confirmed the presence of limosa, melanuroides, and bohaii subspecies at the study sites. Thus, indeed, Black-tailed Godwits subspecies, despite having distinct breeding ranges, exhibit (partially) overlapping non-breeding ranges in Asia. The subspecies composition differed significantly between sites, with limosa and bohaii dominating in Tanguar Haor and melanuroides in Nijhum Dweep. Of the 21 individuals that were tracked to their breeding grounds, 18 migrated to the expected breeding range of their respective subspecies. However, one bird with a limosa haplotype migrated to a known breeding area of bohaii, whereas two birds with melanuroides haplotypes migrated to the supposed breeding range of limosa. Therefore, while ecological factors at both ends of the flyways may shape the morphological and behavioural differences between Black-tailed Godwit subspecies, their delineations and possible gene flow require further studies.
A first requirement for biological insight, and even for proper management and protection of any species, is to understand the structure of a population, and, in the case of migratory birds, to know the patterns of connectivity between breeding and non-breeding areas (Webster and Marra, 2005; Boulet and Norris, 2006; Lopes et al., 2013). Depending on the species, subspecies may show varying degrees of overlap during breeding and non-breeding seasons. While some species (Red-necked Phalarope Phalaropus lobatus) show sharp migratory divides between breeding populations, migrating along separate routes to distinct non-breeding areas (van Bemmelen et al., 2019), others (e.g. Black-tailed Godwit Limosa limosa, Bar-tailed Godwit Limosa lapponica, Dunlin Calidris alpina, Peregrine Falcon Falco peregrinus, Sandhill Cranes Grus canadensis) show partial or complete overlap in their geographical distribution during (part of) the annual cycle, even among individuals from distinct breeding areas (Piersma et al., 2005; Dixon et al., 2012; Lopes et al., 2013; Ivey et al., 2015; Murray et al., 2018; Lagassé et al., 2020). For geographically distinct subspecies, count and biometric data along their flyways can be pieced together relatively easily to sketch the structure of migration phenology and networks (Dick et al., 1987; Piersma and Jukema, 2002). For subspecies with overlapping distributions, interpreting changes in demography, timing or behaviour at any site requires the identification of each subspecies (Esler, 2000).
Subspecies of several shorebirds, including the near-threatened Black-tailed Godwit, share non-breeding grounds. Black-tailed Godwits have an extensive and fragmented breeding range from Iceland in the west to Chukotka in the far east of Russia, with non-breeding populations in Europe, Africa, the Middle East, China, South East Asia and Australasia (van Gils et al., 2020; Zhu et al., 2021a). Worldwide, four subspecies have been recognized: L. l. islandica, L. l. limosa, L. l. melanuroides, and L. l. bohaii (Prater et al., 1977; Höglund et al., 2009; Zhu et al., 2021a). Along the East Atlantic Flyway, the Icelandic L. l. islandica and nominate subspecies L. l. limosa, distinguished by morphology, show clear differences in breeding distributions, but overlap in staging and non-breeding areas (Masero et al., 2009; Alves et al., 2010; Lopes et al., 2013; Verhoeven et al., 2021; Loonstra et al., 2023). Their co-occurrence in southern Europe correlates with differential habitat use, with L. l. islandica using more coastal habitats than L. l. limosa. Similarly, L. l. melanuroides use more saline habitats than L. l. bohaii in the East Asian–Australasian Flyway (Zhu et al., 2023). As a result, trends in specific behaviors, habitat use and population sizes of Black-tailed Godwits can only be explained by considering the contributions of each subspecies.
A recent study revealed shared non-breeding of L. l. melanuroides and L. l. bohaii subspecies, which co-occur in Southeast Asia and as far south as Australia in the East Asian–Australasian Flyway (Zhu et al., 2023). Consequently, the question arises to what extent different subspecies co-occur at non-breeding sites in South Asia, particularly in the Ganges-Brahmaputra-Meghna Delta in Bangladesh—the world's largest river delta. Large flocks (~3000) of Black-tailed Godwits are regularly observed in the delta (Das et al., 2022; Khan, 2018), situated at the conjunction of the Central Asian Flyway and the East Asian–Australasian Flyway. Some studies (Ali and Ripley, 1983; Rasmussen and Anderton, 2005; Sangha, 2021) suggested the occurrence of both limosa and melanuroides. But Bamford et al. (2008) have suggested that the melanuroides subspecies (including the then yet-to-be-discovered bohaii subspecies) is confined to the East Asian–Australasian Flyway, thus excluding India and Bangladesh. Hence, Bangladeshi Black-tailed Godwits would mostly be L. l. limosa. Zhu (2023), however, suggested that both Asian subspecies, L. l. melanuroides and the newly discovered L. l. bohaii, as well as the western Eurasian L. l. limosa, could share non-breeding grounds in Bangladesh (Fig. 1).
To unveil the subspecies composition and breeding origins of Black-tailed Godwits in the Ganges-Brahmaputra-Meghna Delta, we employed a combination of biometric, genetic and tracking methods. Expecting multiple subspecies, we collected biometric data to compare the morphology of Black-tailed Godwits from the Bangladeshi non-breeding population to reference data from known subspecies. To ensure sufficient sampling and gain insights into differential habitat use, we selected two ecologically distinct habitats known to host the largest concentrations of non-breeding Black-tailed Godwits in Bangladesh: Nijhum Dweep National Park, a tidal coastal habitat with brackish water, and Tanguar Haor (‘backmarsh’), a seasonal freshwater floodplain. We also hypothesized that different subspecies of Black-tailed Godwits differentially use coastal and inland sites, freshwater-preferring subspecies dominating Tanguar Haor and coastal-preferring subspecies dominating in Nijhum Dweep.
Field expeditions were conducted in Nijhum Dweep National Park (22°03′51.4″ N, 91°00′03.4″ E) during December 2021, January 2022, February 2023, and April 2023. In Tanguar Haor (25°08′57.56″ N, 91°03′41.42″E), expeditions were conducted in February 2022, and again from February to March 2023 (Appendix Table S1). The two study sites, approximately 300 km apart, are part of the East Asian–Australasian Flyway Site and are known for hosting large numbers (~3000) of Black-tailed Godwits during the non-breeding season (Das et al., 2022; Alam et al., 2023).
The Nijhum Dweep National Park covers an area of 16,352 ha and is located in the Meghna Estuary of the Bay of Bengal at the central coast of Bangladesh (Fig. 2). The park is part of the Ganges-Brahmaputra-Meghna Delta. The delta has been recognized as an Important Bird and Biodiversity Area (IBA) by BirdLife International. The area has a semidiurnal tidal regime and is dynamic in nature, with sediment accretion and erosion constantly reshaping and resizing the islands (Hossain et al., 2016; see further details in Das et al., 2022).
Tanguar Haor (Fig. 2), a Ramsar Site in Bangladesh, is recognized as one of the important freshwater wetlands in Asia for waterbirds (Alam et al., 2023). The haor is located at Sunamganj district in northeastern Bangladesh, near the border (ca. 1.5 km) with Garo Hills of Meghalaya state of India. The haor falls within the catchment area of the world's highest annual rainfall centered in Cherrapunji, India. With an average annual rainfall of over 5000 mm in haor region during the rainy season (May–September), the entire area becomes submerged. During the dry season (October–April), water levels fall from 6–10. m to 2–6. m, resulting in numerous shallow waterbodies (mean ± SD, 7.34 ± 1.88 m), channels, reedbeds, other wetland grasses, and emergent herbage (IUCN Bangladesh, 2015).
During New Moon (ca. seven nights), we placed three-shelved mist nets along the flightpaths to a high-tide night roost site in Nijhum Dweep and at a night roost site in Tanguar Haor. To ensure the safety of captured birds, we checked the nets every 30–40. min and promptly extracted any birds. The mist nets were closed during inclement weather, such as heavy wind and rain, or during peak high-tide hours when high water levels made monitoring unsafe or impractical. In 2021–2022., we placed noose traps at both sites during daytime in frequent foraging areas of Black-tailed Godwits. In total, 93 Black-tailed Godwits were captured, only one of which was captured by noose trap. Captured Black-tailed Godwits received three color bands on one tibia (unique combination of blue, lime, red and yellow) and two yellow flags on the other tibia according to the East Asian–Australasian Flyway protocol. We measured the length of bill (exposed culmen), total head, tarsus and tarsus-toe to the nearest 0.1 mm, and the flattened wing length (chord) was measured to the nearest 1 mm (Zhu et al., 2021b). We weighed the bird to the nearest 1.0 g with an electronic scale. The birds’ ages were determined on the basis of wear and color of the primary feathers (Prater et al., 1977).
We deployed two models of solar-powered GPS–GSM transmitters (Hunan Global Messenger Technology Co., Ltd., China) using a leg-loop harness of 2-mm Dyneema rope (Verhoeven et al., 2021). Tags of 4.5 g were deployed in 2021–2022. (n = 20) and 2022–2023. (n = 13), and 6.5 g tags were deployed in 2022–2023. (n = 21). To increase the charging potential of the 4.5 g tags, we glued a 4 mm thick neoprene pad to their base in 2022–2023.. We selected individuals for tagging based on their body weight and deployed a tag only if the combined weight of tag and harness did not exceed 5% of the total body mass of the bird (Amelon et al., 2009; Barron et al., 2010). The average body mass of captured individuals was 216.3 ± 39.9 g. The weight of deployed tags ranged from 1.7% to 4.3% of the birds' body mass. To ensure safety, we kept tagged birds in a separate, quiet cage to recover from handling stress, and ensured they did not show abnormal behaviour from tagging. We released them at first light in the morning if they were healthy. Transmitters weighing 4.5 g were programmed to take positions at intervals of 6–24. h while those weighing 6.5 g were programmed to take positions at intervals of 3–24. h, depending on battery levels.
Blood samples of 20–60. μL were taken from the brachial vein and stored in 96% ethanol at −20 ℃ for subsequent molecular DNA analysis. For molecular sexing, we used the sexing primer pair 2602F and 2669R following the methodology described by van der Velde et al. (2017). For subspecies identification, we used the primer pair L130 and H772 to sequence a 642 base pair (bp) fragment of the Control Region (CR) in the mitochondrial DNA; for this we applied the methodology of Zhu et al. (2021b).
Sequence quality check and processing were performed using Geneious prime 2023.1.1 (Geneious Prime, 2023.1.1, https://www.geneious.com). We aligned forward and reverse CR sequences blindly, i.e. before assigning the field ID, yielding a single unambiguous consensus sequence for each sample. One sample had to be excluded as pairwise alignment of forward and reverse sequence was not possible due to poor sequence quality. Next, we created an alignment of 287 sequences combining 92 sequences (GenBank accession numbers PP975154−PP975245) from this study and 195 sequences (GenBank accession numbers MT302861−MT303055) from Zhu et al. (2021b). We reproduced the haplotype network of Zhu et al. (2021b) for reference, and combined it with our sequences to visualize the identity of Black-tailed Godwits subspecies captured from Nijhum Dweep and Tanguar Haor in Bangladesh. The R (v.4.3.1) package Pegas (Paradis et al., 2023) was used to visualize the haplotype network and also to determine haplotypes and their frequency of occurrence in our samples (further details in Zhu et al., 2021b). In the process, we made sure by repeated checking that we did not make any error in tracing IDs from sequence to field ID.
We imported tracking data from Movebank (http://www.movebank.org; Movebank ID: 1862069405) using the move package (Kranstauber et al., 2023) in R. Next, we inspected tracks for large-scale outliers. We did not find any outlier in our dataset based on geographical domain limits or speed criteria. To identify intended breeding destinations of Black-tailed Godwits, we then segmented each individual track into consecutive migration cycles from April in year i through March in year i + 1, and retained only those migration cycles during which a Black-tailed Godwit travelled at least 3000 km north of Nijhum Dweep (22°11′31.2″ N, 91°05′34.8″ E), far enough to reach the nearest breeding areas of any subspecies (n = 21 cycles of 19 birds).
Based on inspection of the data, we classified birds’ intended breeding destinations as the locations they reached furthest away from Nijhum Dweep, and we verified that the birds spent at least 30 days at those locations during the known breeding season (April–July) to allow enough time for settlement in breeding areas and a (failed) breeding. If an individual spent <30 days at a location >3000 km north of Nijhum Dweep, we did not consider the location as intended breeding destination. Furthermore, we manually checked each track to verify there were no other long staging events in potential breeding areas. We then verified whether the intended breeding destinations were situated within the presumed breeding distributions for each subspecies (Zhu et al., 2022; BirdLife International, 2023).
Subspecies composition between study sites: We conducted a Chi-square test across all birds captured at our two study sites to determine whether there were any differences in site preference between subspecies. In doing so, we accounted for the possibility that site preferences might differ between subspecies as has been recorded between the icelandica and limosa subspecies in the East Atlantic Flyway (Alves et al., 2010). We also tested site-preferences separately for males and females.
Subspecies and sex differences in biometrics: We aimed to test whether subspecies and sex differences in the biometrics of Bangladeshi Black-tailed Godwits were consistent with differences reported in previous studies. To do this, we implemented Generalized Linear Models (GLM) for each biometric (bill length, head + bill length, wing length, tarsus length, tarsus + toe length) using subspecies, sex and an interaction effect as independent variables.
Additionally, we were interested in testing whether biometrics of Bangladeshi Black-tailed Godwits differ from the biometrics of the same subspecies and sex measured in the Netherlands (L. l. limosa), China (L. l. bohaii and L. l. melanuroides), and Australia (L. l. melanuroides) by Zhu et al. (2020). To do this, we focused on bill, wing and tarsus length which were measured in consistent way by different researchers. We excluded head + bill and tarsus + toe measurements because we included nail in tarsus-toe measurements, whereas Zhu et al. (2020) did not. For each subspecies, we then constructed a set of three candidate GLM models for the bill, wing and tarsus length: a single effect model for sex, a model including additive effects of sex and study, and a model including the interaction effect of sex and study. We then used Aikaike's Information Criterion (AIC) to identify the best models. In case we found models with comparable performance (ΔAIC < 2), we chose the simplest model following the principle of parsimony.
For each GLM we assumed a Gaussian error distribution, and used a Shapiro–Wilk test to verify that model residuals satisfied the assumption of normality. This was the case for most models. In a few cases the assumption of normality was satisfied once we removed one to two outliers.
We obtained a 463 bp sequence alignment of the mtDNA CR from 287 individuals, combining 92 individuals from Bangladesh with 195 from Zhu et al. (2021b), to construct a haplotype network. The network analysis produced 44 mtDNA haplotypes, 36 of which matched those reported by Zhu et al. (2021b). Eight haplotypes were new. The four major haplotype clusters—cluster I for L. l. islandica, II for L. l. limosa, III for L. l. bohaii, and IV for L. l. melanuroides—were reproduced as in Zhu et al. (2021b). Samples from Nijhum Dweep and Tanguar Haor occurred in haplotype clusters II, III, and IV, but did not occur in haplotype cluster I (Fig. 3).
All three subspecies occurred in both Nijhum Dweep and Tanguar Haor but the composition differed significantly between sites, with sex being a key factor. The melanuroides subspecies represented a higher proportion of captures at Nijhum Dweep than at Tanguar Haor during both non-breeding seasons (Table 1; Fig. 4, χ2 = 54.11, df = 2, p = 1.779−12). Notably, neither limosa males nor bohaii females were present among the 64 godwits captured in Nijhum Dweep over two non-breeding seasons (Table 1). A Chi-square test showed a significant association between study sites and each sex (Table 1, χfemale2 = 31.58, df = 2, p < 0.0001; χmale2 = 25.48, df = 2, p < 0.0001).
Study site | Subspecies | Non-breeding season | Female | Male |
Nijhum Dweep | L. l. limosa | 2021–2022. | 1 | 0 |
2022–2023. | 1 | 0 | ||
L. l. melanuroides | 2021–2022. | 11 | 8 | |
2022–2023. | 24 | 17 | ||
L. l. bohaii | 2021–2022. | 0 | 1 | |
2022–2023. | 0 | 1 | ||
Tanguar Haor | L. l. limosa | 2021–2022. | 4 | 3 |
2022–2023. | 1 | 4 | ||
L. l. melanuroides | 2021–2022. | 2 | 1 | |
2022–2023. | 1 | 1 | ||
L. l. bohaii | 2021–2022. | 3 | 2 | |
2022–2023. | 4 | 2 | ||
Total | 52 | 40 |
Although measurement ranges overlapped in all cases, individuals of the limosa subspecies were on average larger than melanuroides and bohaii individuals in all measurements. In addition, bohaii had longer bills than melanuroides, while their wing and tarsus lengths were similar. Again as expected, in each of the three subspecies females were larger than males (Fig. 5, Table 2, Appendix Table S2). We found significant deviations in at least one body measurement for each of the three subspecies among the Bangladeshi birds compared to measurements elsewhere (Appendix Fig. S1, Tables S3 and S4). The Bangladeshi bohaii population is smaller in all three body size measurements. However, male individuals of limosa measured in Bangladesh were larger in all three measurements.
Bill (mm) | Total head (mm) | Wing (mm) | Tarsus (mm) | Tarsus-toe (mm) | n | ||
♀ | limosa | 108.89 ± 8.41 | 143.69 ± 9.48 | 216.86 ± 9.81 | 79.21 ± 5.95 | 131.00 ± 7.02 | 7 |
bohaii | 104.76 ± 6.82 | 137.96 ± 4.90 | 203.86 ± 5.58 | 71.07 ± 3.54 | 116.71 ± 5.88 | 7 | |
melanuroides | 86.40 ± 4.90 | 121.10 ± 5.35 | 202.03 ± 5.83 | 70.29 ± 3.84 | 116.04 ± 4.86 | 38 | |
♂ | limosa | 93.79 ± 7.99 | 130.11 ± 8.92 | 209.14 ± 13.26 | 74.67 ± 8.60 | 124.00 ± 11.47 | 7 |
bohaii | 85.67 ± 6.26 | 119.85 ± 5.66 | 197.67 ± 4.68 | 63.57 ± 2.05 | 108.67 ± 5.82 | 6 | |
melanuroides | 76.32 ± 3.40 | 110.00 ± 4.55 | 193.04 ± 5.42 | 63.67 ± 3.11 | 107.17 ± 6.36 | 27 |
We analyzed breeding destinations based on 21 northward migration tracks from 19 individuals. Among the remaining tagged individuals, four did not meet the criteria, nine stayed in Bangladesh, five died, and 17 tags stopped transmitting signals. Nineteen individuals migrated further than 3000 km from Nijhum Dweep, including one individual with a haplotype of the bohaii subspecies, five limosa individuals and 13 melanuroides individuals (Fig. 6). Most of these migrated to breeding regions corresponding with their haplotype, but one limosa individual and two melanuroides individuals did not (Fig. 6). One limosa migrated to the Sakha Republic of Russia which is known as a breeding region of L. l. bohaii. Two melanuroides migrated to the Ulaangom area in Mongolia, supposedly a breeding region of the limosa subspecies (Fig. 6). The two individuals (one limosa and one melanuroides) that were tracked across two consecutive migration cycles returned to the same breeding destination in each cycle.
We confirmed the presence of three subspecies of Black-tailed Godwits—limosa, bohaii and melanuroides—in both coastal and inland freshwater wetlands of Bangladesh. The mtDNA haplotype network confirmed the divergence among the subspecies islandica, limosa, bohaii, and melanuroides reported by Zhu et al. (2021b). We disprove previous assumptions that melanuroides subspecies is restricted to the East Asian–Australasian Flyway (Bamford et al., 2008). In fact, the co-occurrence of the bohaii, limosa, and melanuroides subspecies of Black-tailed Godwits in Bangladesh highlights the region as a unique non-breeding junction for the species. It provides empirical evidence of the connection between birds from two global migratory flyways: the Central Asian Flyway and the East Asian–Australasian Flyway.
Body size variation within a species is a long-established basis for differentiating subspecies (Hamilton, 1961). The variations in body size among subspecies serve as indicators of their ecological adaptations and likely encompass an array of behavioral, physiological, and ecological nuances (Grant, 1968; Olson et al., 2009; Töpfer, 2018; Zimova et al., 2023). These ecological adaptations may also facilitate niche partitioning (Grant and Grant, 1979; Bellefontaine and Hamilton, 2023), where each Black-tailed Godwit subspecies exhibits unique foraging strategies to exploit habitats within the wetlands of Bangladesh and therefore can coexist as they minimize direct competition for resources. For instance, the larger and longer-billed limosa subspecies may access prey buried deeper in the mud of any given area, or forage in areas with deeper waters, while the smaller melanuroides subspecies might specialize in shallower waters or different food resources. The observed body size differences of Bangladeshi bohaii and limosa subspecies, compared to populations in Australia, China and the Netherlands, may indicate morphological adaptations to specific conditions or resources in the Ganges-Brahmaputra-Meghna Delta. These findings highlight our study sites as valuable systems for future research, combining long-term ecological monitoring and genomic studies, to explore how ecological conditions might influence body size and shape through phenotypic plasticity or microevolution (Chakraborty et al., 2023; Fordyce, 2006; Zhu et al., 2020).
The difference in subspecies composition between capture sites aligned with our predictions, with limosa and bohaii subspecies dominating in Tanguar Haor and melanuroides in Nijhum Dweep. Our sampling periods may have influenced on the observed catch composition between sites, as shorebird numbers at a site vary in the course of a non-breeding season. The fact that melanuroides dominated catches at Nijhum Dweep in December and January (2021–2022.) as well as in February and April (2022–2023.) suggests the site differences are consistent (Fig. 4) throughout the non-breeding season and minimally impacted by our sampling periods. Our inspection of GPS tracking data from the non-breeding seasons revealed that while some godwits moved within Bangladesh, no movement between Tanguar Haor and Nijhum Dweep was detected yet (Appendix Fig. S2). The absence of limosa males and bohaii females in Nijhum Dweep is striking, but in view of the limited sampling it is too early to claim that male limosa and female bohaii prefer the freshwater habitat of Tanguar Haor over the coastal region of Nijhum Dweep. Nevertheless, the observation suggests social (and possibly habitat) segregation between the sexes (Catry et al., 2006; Conradt, 2006) in at least two subspecies. Differential habitat use between sexes seems to occur more commonly at local scales (Selander, 1966; Durell, 2000) than at large spatial scales (Catry et al., 2006). Sex-specific space use patterns have been observed in local studies of Bar-tailed Godwits (Smith and Evans, 1973; Zwarts, 1988), Eurasian Curlew (Numenius phaeopus) (Zwarts, 1988; Townsend et al., 2012) and Curlew Sandpiper (Calidris ferruginea) (Puttick, 1981), but not in Hudsonian Godwits (L. haemastica) (Basso et al., 2024) and Black-tailed Godwits (Alves et al., 2013; but see Masero et al., 2009). Sexual segregation is often explained by body size dimorphism that influences social dominance and specialization-based niche segregation (Catry et al., 2006). Bill length is the most dimorphic body feature (Zhu et al., 2020) between sexes of all four subspecies of Black-tailed Godwits that might lead to differential space and habitat use during non-breeding season (Catry et al., 2006; Conradt, 2006). Yet, the four (sex and/or subspecies) categories do not assort to Nijhum Dweep according to bill length. Relatively shorter-billed bohaii males (on average eight mm shorter than that of limosa males) were present in our catches while larger-billed bohaii females were absent (Table 1, Table 2). We suggest that limosa and bohaii subspecies are sexually segregated along the coast, but not at Tanguar Haor. A nontidal site, Tanguar Haor provides opportunities to forage longer during the few months (January–March) when the water level is low enough. At this point, we suggest that the differential use of sites and habitats between subspecies and sexes offers an interesting eco-evolutionary puzzle. Solving this puzzle will require the targeted collection of information on food availability across different sites and habitats to infer how morphological variation might be associated with resource availability and habitat conditions.
The movements of Bangladeshi Black-tailed Godwits GPS-tracked in this study are mostly consistent with the currently recognized breeding ranges for each of the three subspecies (van Gils et al., 2020; Zhu et al., 2021a; BirdLife International, 2023), but may indicate incomplete knowledge in the areas of potential geographical overlap. Nevertheless, we found three cases where an individual's breeding location did not match the expected haplotype, which could change our perspective on this species' population genetics. Theoretically, even a very low rate of immigration from neighboring populations should, over time, prevent significant genetic differentiation of populations (Slatkin, 1985). If we were to detect three apparent “dispersal events” in a sample of just 21 tracked individuals, it would suggest a much higher rate of exchange among populations, which seemingly contradicts the clear separation of mtDNA haplotypes found among limosa, bohaii, and melanuroides (Zhu et al., 2021b). At least three possible scenarios could explain this apparent contradiction. First, it is possible that there is a greater mtDNA diversity overlap between subspecies than observed in the previous study, such that breeding location for some individuals is a better indication of subspecies membership than haplotype. Second, the high rate of exchange could be a relatively recent development (e.g., tens or hundreds of years), such that the homogenizing effect of immigration is yet to be detected in mtDNA. Contemporary changes in migration patterns, associated with habitat loss or climate change, have been predicted in this species and similar shorebirds (Bom et al., 2024; Lisovski et al., 2024). Third, these unexpected tracks might not represent true dispersal among populations. For example, ventures into a neighboring breeding area may be exploratory rather than actual breeding attempts, or may not lead to successful breeding, either due to assortative mating or selection against immigrants (Nosil et al., 2005), in which case there is no effective dispersal. The co-occurrence of three Black-tailed Godwits subspecies in the Ganges-Brahmaputra-Meghna Delta in Bangladesh provides an opportunity for breeding populations to mix in the non-breeding season, with possible consequences for migration and breeding. In view of the evidence that young godwits learn the first southward migration such as from conspecifics (Loonstra et al., 2023), the “dispersal events” may represent young birds who joined flocks of another subspecies during the first northward migrations. However, three dispersed birds were aged as adult during sampling. The rates of dispersal and gene flow among these subspecies could be further investigated through a combination of multi-year tracking and higher-resolution genomic analysis.
The use of different areas by different subspecies and sexes has conservation implications. If individuals of a subspecies concentrate on specific habitat patches or diets, the impact of habitat loss due to climate change or anthropogenic reasons will differ between subspecies. Habitat segregation between subspecies during the non-breeding period might be more common among migratory species than currently recognized. Our results thus underscore the need to identify the extent to which populations and subspecies mix or segregate along and across flyways. Without such information, conservation measures risk neglecting or confusing certain sex and subspecies, or the impacts of habitat loss and climate change can be underestimated. Therefore, understanding how overlapping subspecies segregate in habitat use can help setting conservation priorities and developing targeted habitat management strategies.
Delip K. Das: Writing – review & editing, Writing – original draft, Software, Project administration, Methodology, Investigation, Funding acquisition, Formal analysis, Data curation, Conceptualization. Wouter M.G. Vansteelant: Writing – review & editing, Methodology, Formal analysis. Bingrun Zhu: Writing – review & editing, Visualization. Shariful Islam: Project administration, Data curation. Naim Khandakar: Project administration, Data curation. Marco van der Velde: Formal analysis. Chris J. Hassell: Resources. Jesse R. Conklin: Writing – review & editing. Pierrick Bocher: Resources, Funding acquisition, Data curation. Jos C.E.W. Hooijmeijer: Writing – review & editing, Resources, Data curation. Yvonne I. Verkuil: Writing – review & editing, Software, Methodology, Formal analysis. Theunis Piersma: Writing – review & editing, Supervision, Funding acquisition, Conceptualization.
The authors declare that they have no known competing financial interests or personal relationships that could have appeared to influence the work reported in this paper.
We acknowledge the financial support from the Embassies of France and the Kingdom of the Netherlands in Dhaka for financial support of the field expeditions, and the personal support of Folkert G.J. de Jager and Ambassador Anne van Leeuwen at the Dutch embassy. We also thank Tienke Koning for great help with fundraising. Delip K. Das acknowledges the personal study grant from the Bangabandhu Science and Technology Fellowship Trust. We are thankful to Bangladesh Forest Department for the research permit and to Bangladesh bird club and IUCN Bangladesh for logistical support in the field. We thank Dirk Smit (former Corporate Chief Scientist, Shell International) for financing the transmitters. We thank Mohammad Shamsuddoha, Mohamed Henriques and Taylor Craft for helping to produce maps and our volunteers and field assistants for the challenging field work: Soab Ali, Sabit Hasan, Al Jubayet, Prithul, Atikul Islam Mithu, Ashikur Rahman Zisan, Golam Sakline Sathil, Taijul Islam and Rafikul Islam. For the Australian dataset, we thank the many dedicated volunteers who participated in the years of fieldwork and Broome Bird Observatory for logistical support. CJH was variously funded by WWF-Netherlands, BirdLife Netherlands, the Spinoza Premium 2014 awarded by the Netherlands Organization for Scientific Research (NWO) to Theunis Piersma, and the MAVA Foundation, Switzerland. We acknowledged the Yawuru People via the offices of Nyamba Buru Yawuru Limited for permission to catch birds on the shores of Roebuck Bay, traditional lands of the Yawuru people. We thank the editor, two anonymous reviewers, and Taej Mundkur for their constructive feedback.
Supplementary data to this article can be found online at https://doi.org/10.1016/j.avrs.2025.100226.
Ali, S., Ripley, D., 1983. Handbook of the Birds of India and Pakistan, Compact. Oxford University Press, Delhi.
|
Amelon, S., Dalton, D., Millspaugh, J., Wolf, S., 2009. Radiotelemetry: techniques and analysis. In: Kunz, T.H., Parsons, S. (Eds.), Ecological and Behavioral Methods for the Study of Bats. Johns Hopkins University Press, Baltimore, USA, pp. 57–77.
|
Bamford, M., Watkins, D., Bancroft, W., Tischler, G., Wahl, J., 2008. Migratory shorebirds of the East Asian–Australasian flyway: population estimates and internationally important sites. Wetlands International – Oceania.
|
Catry, P., Phillips, R.A., Croxall, J.P., 2006. Sexual segregation in birds: patterns, processes and implications for conservation. In: Ruckstuhl, K., Neuhaus, P. (Eds.), Sexual Segregation in Vertebrates. Cambridge University Press, UK, pp. 351–378.
|
Conradt, L., 2006. Definitions, hypotheses, models and measures in the study of animal segregation. In: Ruckstuhl, K., Neuhaus, P. (Eds.), Sexual Segregation in Vertebrates. Cambridge University Press, Cambridge, UK, pp. 11–32.
|
Das, D.K., Khandakar, N., Sultana, I., Islam, S., Ali, MdS., Galib, A.J., et al., 2022. Site use by non-breeding black-tailed godwits at Nijhum Dweep national park, Bangladesh. Wader Study 129, 14–21.
|
Dick, W.J.A., Piersma, T., Prokosch, P., 1987. Spring migration of the siberian knots Calidris canutus canutus: results of a co-operative wader study group project. Ornis Scand. 18, 5–16.
|
IUCN Bangladesh, 2015. Tanguar Haor Management Plan Framework and Guidelines. IUCN Bangladesh, Dhaka, Bangladesh.
|
Khan, M.M.H., 2018. Photographic Guide to the Wildlife of Bangladesh. Arannayk Foundation, Dhaka, Bangladesh.
|
Lagassé, B., Lanctot, R., Barter, M., Brown, S., Chiang, C.-Y., Choi, C.-Y., et al., 2020. Dunlin subspecies exhibit regional segregation and high site fidelity along the East Asian-Australasian Flyway. Condor 122, 1–15.
|
Masero, J.A., Santiago-Quesada, F., Sanchez-Guzman, J.M., Abad-Gomez, J.M., Villegas, A., Albano, N., 2009. Geographical origin, return rates, and movements of the near-threatened Black-tailed Godwits Limosa limosa staying at a major stopover site of Iberia. ARDEOLA 56, 253–258.
|
Nosil, P., Vines, T.H., Funk, D.J., 2005. Perspective: reproductive isolation caused by natural selection against immigrants from divergent habitats. Evolution 59, 705–719.
|
Paradis, E., Jombart, T., Kamvar, Z.N., Knaus, B., Schliep, K., Potts, A., et al., 2023. Pegas: Population and Evolutionary Genetics Analysis System.
|
Piersma, T., Rogers, D.I., Gonzalez, ´ P.M., Zwarts, L., Niles, L.J., Lima, I. de, et al., 2005. Fuel storage rates before northward flights in Red Knots worldwide. In: Greenberg, R., Marra, P.P. (Eds.), Birds of Two Worlds: the Ecology and Evolution of Migration. Johns Hopkins University Press, Baltimore, Maryland, pp. 262–274.
|
Prater, A.J., Prater, T., Marchant, J., Vuorinen, J., 1977. Guide to the identification and ageing of holarctic waders, BTO guide. British Trust for Ornithology.
|
Rasmussen, P.C., Anderton, J.C., 2005. Birds of South Asia: the Ripley Guide. Lynx Edicions, Barcelona, Spain.
|
Sangha, H.S., 2021. Waders of the Indian Subcontinent. Harkirat Singh Sangha, Jaipur.
|
Slatkin, M., 1985. Gene flow in natural populations. Annu. Rev. Ecol. Systemat. 16, 393–430.
|
Töpfer, T., 2018. Morphological variation in birds: plasticity, adaptation, and speciation. In: Tietze, D.T. (Ed.), Bird Species, Fascinating Life Sciences. Springer International Publishing, Cham, pp. 63–74.
|
van Bemmelen, R.S.A., Kolbeinsson, Y., Ramos, R., Gilg, O., Alves, J.A., Smith, M., et al., 2019. A migratory divide among Red-Necked Phalaropes in the Western Palearctic reveals contrasting migration and wintering movement strategies. Front. Ecol. Evol. 7, 86
|
van der Velde, M., Haddrath, O., Verkuil, Y.I., Baker, A.J., Piersma, T., 2017. New primers for molecular sex identification of waders. Wader Study 124, 147–151.
|
van Gils, J., Wiersma, P., Christie, D., Garcia, E., Boesman, P.F.D., 2020. Black-tailed Godwit (Limosa limosa). In: Billerman, S.M., Keeney, B.K., Rodewald, P.G., Schulenberg, T.S. (Eds.), Birds of the World. Cornell Lab of Ornithology.
|
Webster, M.S., Marra, P.P., 2005. The importance of understanding migratory connectivity and seasonal interactions. In: Greenberg, R., Marra, P.P. (Eds.), Birds of Two Worlds: The Ecology and Evolution of Migration. Johns Hopkins University Press, Baltimore, Maryland, pp. 199–209.
|
Zhu, B.R., 2023. Asian Black-tailed Godwits as New Players in Comparative Approaches to Morphology, Sexual Size Dimorphism and Flyway Ecology. Doctoral Thesis. University of Groningen, The Netherlands.
|
Zhu, B.R., Verhoeven, M.A., Hassell, C.J., Leung, K.K.-S., Dorofeev, D., Ma, Q., et al., 2023. Predicting the non-breeding distributions of the two Asian subspecies of Black-tailed Godwit using morphological information. Avian Res. 14, 100069
|
Zimova, M., Weeks, B.C., Willard, D.E., Giery, S.T., Jirinec, V., Burner, R.C., et al., 2023. Body size predicts the rate of contemporary morphological change in birds. Proc. Natl. Acad. Sci. U.S.A. 120, e2206971120
|
Zwarts, L., 1988. Numbers and distribution of coastal waders in Guinea-Bissau. Ardea 76, 42–55.
|
Study site | Subspecies | Non-breeding season | Female | Male |
Nijhum Dweep | L. l. limosa | 2021–2022. | 1 | 0 |
2022–2023. | 1 | 0 | ||
L. l. melanuroides | 2021–2022. | 11 | 8 | |
2022–2023. | 24 | 17 | ||
L. l. bohaii | 2021–2022. | 0 | 1 | |
2022–2023. | 0 | 1 | ||
Tanguar Haor | L. l. limosa | 2021–2022. | 4 | 3 |
2022–2023. | 1 | 4 | ||
L. l. melanuroides | 2021–2022. | 2 | 1 | |
2022–2023. | 1 | 1 | ||
L. l. bohaii | 2021–2022. | 3 | 2 | |
2022–2023. | 4 | 2 | ||
Total | 52 | 40 |
Bill (mm) | Total head (mm) | Wing (mm) | Tarsus (mm) | Tarsus-toe (mm) | n | ||
♀ | limosa | 108.89 ± 8.41 | 143.69 ± 9.48 | 216.86 ± 9.81 | 79.21 ± 5.95 | 131.00 ± 7.02 | 7 |
bohaii | 104.76 ± 6.82 | 137.96 ± 4.90 | 203.86 ± 5.58 | 71.07 ± 3.54 | 116.71 ± 5.88 | 7 | |
melanuroides | 86.40 ± 4.90 | 121.10 ± 5.35 | 202.03 ± 5.83 | 70.29 ± 3.84 | 116.04 ± 4.86 | 38 | |
♂ | limosa | 93.79 ± 7.99 | 130.11 ± 8.92 | 209.14 ± 13.26 | 74.67 ± 8.60 | 124.00 ± 11.47 | 7 |
bohaii | 85.67 ± 6.26 | 119.85 ± 5.66 | 197.67 ± 4.68 | 63.57 ± 2.05 | 108.67 ± 5.82 | 6 | |
melanuroides | 76.32 ± 3.40 | 110.00 ± 4.55 | 193.04 ± 5.42 | 63.67 ± 3.11 | 107.17 ± 6.36 | 27 |