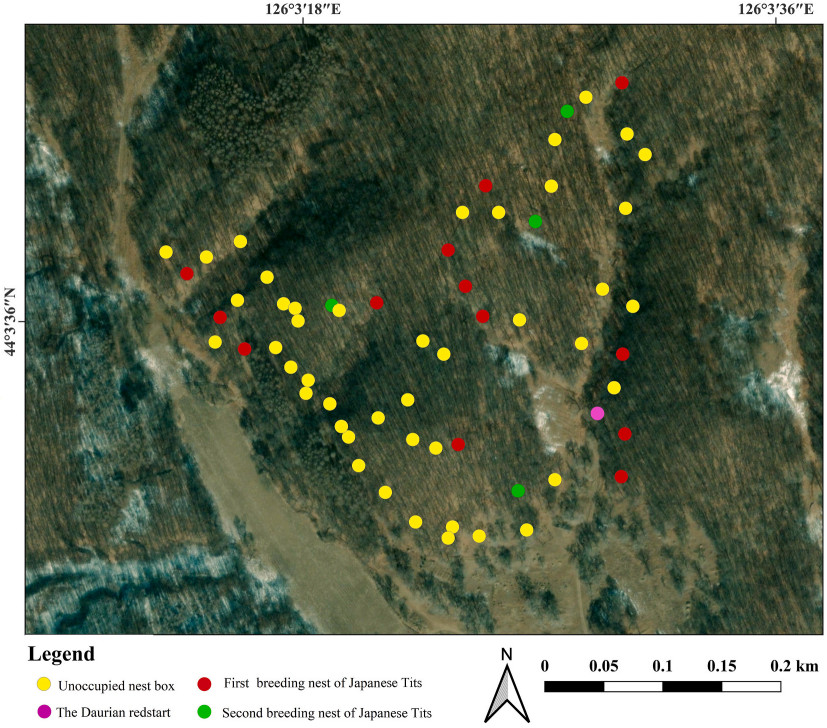
Citation: | Xudong Li, Jiangping Yu, Dake Yin, Longru Jin, Keqin Zhang, Li Shen, Zheng Han, Haitao Wang. 2024: Does social information affect the settlement decisions of resident birds in their second breeding attempt? A case study of the Japanese Tit (Parus minor). Avian Research, 15(1): 100198. DOI: 10.1016/j.avrs.2024.100198 |
Individuals may gather information about environmental conditions when deciding where to breed in order to maximize their lifetime fitness. They can obtain social information by observing conspecifics and heterospecifics with similar ecological needs. Many studies have shown that birds can rely on social information to select their nest sites. The location of active nests and the reproductive success of conspecifics and heterospecifics can provide accurate predictions about the quality of the breeding habitat. Some short-lived species can facultatively reproduce two and/or more times within a breeding season. However, few studies have focused on how multiple-brooding individuals select nest sites for their second breeding attempts. In this study, we use long-term data to test whether the Japanese Tit (Parus minor) can use social information from conspecifics and/or heterospecifics (the Eurasian Nuthatch Sitta europaea, the Daurian Redstart Phoenicurus auroreus and the Yellow-rumped Flycatcher Ficedula zanthopygia) to select a nest site for the second breeding attempt. Our results showed that the nest boxes occupied by tits on their second breeding attempt tended to be surrounded by more breeding conspecific nests, successful first nests of conspecifics, and fewer failed first nests of conspecifics than the nest boxes that remained unoccupied (the control group). However, the numbers of breeding heterospecific nests, successful heterospecific nests, and failed heterospecific nests did not differ between the nest boxes occupied by tits on their second breeding attempt and the unoccupied nest boxes. Furthermore, the tits with local successful breeding experience tended to choose areas with more successful first nests of conspecifics than those without successful breeding experience. Thus, we suggest that conspecifics' but not heterospecifics’ social information within the same breeding season is the major factor influencing the nest site selection of Japanese Tits during second breeding attempts.
When animals make decisions that will impact their lifetime fitness, such as where to breed (Melles et al., 2003; Acker et al., 2017; Brandl et al., 2018; Podofillini et al., 2018), what to forage (García-Navas and Sanz, 2010; Firth et al., 2016), and with whom to mate (Jones and Ratterman, 2009; Hegyi et al., 2015), natural selection should promote behavioral mechanisms that will help reduce uncertainty around these decisions (Clark and Shutler, 1999). To increase the likelihood of making the best choices, they often collect multiple sources of information about environmental conditions (Schmidt et al., 2010; Kearns and Rodewald, 2013; Tolvanen et al., 2020). Individuals may gather information by sampling their environment or through their personal experience, termed “private information” (Citta and Lindberg, 2007; Kearns and Rodewald, 2013; Frommhold et al., 2019; Berg and Eadie, 2020). Moreover, individuals can gather information by observing conspecifics and heterospecifics that have similar ecological requirements, interacting with the environment and/or observing the consequences of their decisions, termed “social information”, which is accessible to all observing individuals (Doligez et al., 2002; Danchin et al., 2004; Dall et al., 2005; Parejo et al., 2005).
Observing and copying the behavior of other individuals may have important implications for the behavior, fitness and development of traditions among animals (Fragaszy and Perry, 2003; Danchin et al., 2004). For example, using social information can enable individuals to save time and energy than collecting private information (Laland, 2004; Dall et al., 2005; Kendal et al., 2005; Webster and Hart, 2006; Webster and Laland, 2008; Brandl et al., 2018). To date, many taxa have been found to utilize social information for the decision-making process, such as birds (Slagsvold and Wiebe, 2011; Thorogood and Davies, 2012; Veit et al., 2015), amphibians (Swanson et al., 2007), fish (van Bergen et al., 2004; Laland et al., 2011), insects (Chittka and Leadbeater, 2005), and mammals (Vale et al., 2014; Page and Bernal, 2020).
In birds, nest site selection is an important component of habitat selection, and the quality of the nest site has been found to strongly impact the breeding success of nesting birds and the survival of nestlings (Martin and Martin, 2001; Kivelä et al., 2014). Most previous studies have focused on individuals using social information gathered from conspecifics/heterospecifics either in previous years or right before settlement to decide whether to attract or avoid their nest site (Forsman et al., 2002; Parejo et al., 2005; Morinay et al., 2021). Generally, presence of earlier breeders can indicate an abundance of resources, a safer environment, and less competition, making it a more favorable choice (Forsman et al., 2007; Jaakkonen et al., 2015). In addition, many birds have been found to visit potential nest sites before they start breeding, a prospecting behavior allowing them to gather social information (Forsman et al., 2007; Ponchon et al., 2015; Valente et al., 2021). Thus, the valuable information from conspecifics or heterospecifics can influence later-arriving individuals seeking optimal nesting sites (Parejo et al., 2007; Slagsvold et al., 2013; Jaakkonen et al., 2015; Ponchon et al., 2015; Valente et al., 2021).
Multiple brooding is a commonly used strategy for short-lived species, as it can maximize both annual and lifetime reproductive success (Lambrechts et al., 2008). At temperate latitudes, some resident species can facultatively reproduce twice within the same breeding season (Lambrechts et al., 2008; Husby et al., 2009). In addition, some of these individuals construct a new nest to reduce nest predation and ectoparasites during the second breeding attempt (Jackson et al., 1989; Styrsky, 2005; Lambrechts et al., 2008; Donahue et al., 2018; Feng et al., 2019). Due to the costs of delayed breeding, the second breeding nest-site selection should be made within a limited time window (Safran, 2004, 2006; Nomi et al., 2018). Using social information is expected to enable individuals to save time and energy for decision-making about nest-site selection (Laland, 2004; Dall et al., 2005; Kendal et al., 2005). Therefore, we could expect individuals to use social information when selecting a secondary breeding site.
In our study area, small second cavity-nesting passerines mainly include the resident Japanese Tit (Parus minor) and the Eurasian Nuthatch (Sitta europaea), the migrant Daurian Redstart (Phoenicurus auroreus) and the Yellow-rumped Flycatcher (Ficedula zanthopygia). Those species have similar niches, such as using nest boxes for breeding, being threatened by the same predators, and feeding mostly on arboreal arthropods. However, their reproductive time is slightly segregated. Among the four species, Japanese Tits and Eurasian Nuthatches are relatively the earliest to breed, occurring at similar times. Daurian Redstarts fall in an intermediate position, while Yellow-rumped Flycatchers breed at the latest time (see Method for details). Japanese Tits are facultative double-brooding birds and approximately 18% of them produce second clutches after successfully raising the first brood. Females initiate second clutches by remating with the same male (Fan et al., 2021) and prefer to switch to a new nest site. However, when Japanese Tits select their second breeding nest site, most pairs of Japanese Tits (from the first breeding attempt), Eurasian Nuthatches, and Daurian Redstarts have already fledged or are near the end of the brooding period, while Yellow-rumped Flycatchers are laying or incubating eggs. In this study, by using long-term data, we analysed whether Japanese Tits use social information of conspecifics and heterospecifics in their second breeding nest site selection.
Previous studies have shown that closely related species of Japanese Tits, adult Great Tits (Parus major) tend to use conspecific cues in their nest site selection (Loukola et al., 2012; Forsman et al., 2014). In addition, individuals who have successful breeding experience may be more familiar with the reproductive situation within the study area, thereby reproductive experience would influence the use of social information (van Bergen et al., 2004; Valone, 2007). Based on the ring recovery data and time points, tit pairs were categorized as second-breeding pairs and late-breeding pairs (no information on the first clutch) in our study area. First, we used a case-control study to investigate the potential implications of social information in Japanese Tits' nest site selection. Second, we examined whether successful breeding experiences influence the use of social information. We predicted that: 1) later breeding pairs and second breeding pairs would end up breeding in areas with more conspecifics and/or heterospecifics; 2) second breeding pairs who have local successful breeding experience would be attracted to nest sites with greater reproductive success of conspecifics and heterospecifics and close to currently breeding conspecifics' and heterospecifics’ nests than late-breeding pairs.
Fieldwork was conducted from March–July over successive years from 2014 to 2021 in an approximately 134.5 ha mixed deciduous forest at Zuojia Nature Reserve (44°1′–45°0′ N, 126°0′–126°8′ E) in Jilin Province, northeastern China (Fig. 1). The study area consisted of nine discrete deciduous forest patches, with nearly homogeneous forest characteristics. The dominant tree species were Quercus mongolica, Tilia mandshurica, Betula davurica, Phellodendron amurense, and Sophora japonica. Since 2004, many artificial nest boxes have been provided for secondary cavity-nesting birds in this area. The Japanese Tit, Eurasian Nuthatch, Daurian Redstart, and Yellow-rumped Flycatcher readily accept artificial nest boxes for breeding, allowing detailed breeding data and the identity of breeders to be collected on a large number of nests. Approximately 400 artificial nest boxes were provided per year in our study area. The nest boxes were distributed among patches and separated by 30–50 m, with 40–50 boxes equally distributed per patch. The average density of nest boxes was 0.24 boxes/ha (range: 0.12–1.58 boxes/ha). All nest boxes were the same size (inner dimensions: 12 cm × 12 cm × 25 cm, entrance diameter approximately 4.5 cm) and had removable roofs. The artificial nest boxes were hung on trees approximately 2.5–3 m above the ground. The tree species on which the nest boxes were hung and the orientation of the box holes were randomly determined.
We removed old nest materials from the artificial nest boxes before the initiation of the breeding season and routinely monitored all nest boxes from late March until mid-July. We monitored nests at least once a week (and more frequently near expected hatch/fledge dates) until the nest fledged or failed. Failed nests were classified as predated (empty nest cup with all contents removed before estimated fledge date; potential predators included snakes or chipmunks) or abandoned (dead nestlings or cold eggs on two or more consecutive visits). The breeding attempts and reproductive data (e.g., the date the first egg was laid, clutch size, hatching date, brood size, and the number of fledglings) of all four bird species (Japanese Tits, Yellow-rumped Flycatchers, Eurasian Nuthatches, and Daurian Redstarts) were recorded as part of routine monitoring (Yu et al., 2017). We surveyed these forest patches and found few natural holes, with almost all breeding Japanese Tits occupying the nest boxes. During the breeding season, an average of 39.8% of nest boxes were occupied (egg-laid) by birds (Japanese Tits 26.3%, Yellow-rumped Flycatchers 8.4%, Eurasian Nuthatches 2.8%, and Daurian Redstarts 2.2%). Among the species which occupy nest boxes for breeding, Japanese Tits are the most defensive in our study area and rarely find their nests usurped by other species. We determined the coordinates of occupied nest boxes using handheld GPS units with an accuracy of ≤10 m. All nest boxes occupied by birds that had initiated egg-laying were considered and included in the data collection.
Almost all adult Japanese Tits breeding in nest boxes in the study area were captured inside boxes during the mid-to-late chick-rearing period. The second breeding attempt was defined as an individual initiating a second clutch following a successful first clutch (at least one fledging leaving the nest) in the same breeding season (Fan et al., 2021). We defined the pairs recaptured from the first breeding attempt as second-breeding pairs (i.e., pairs with successful breeding experience). In addition, we defined pairs that initiated breeding after the first brood of chicks had fledged in the study area (i.e., those with no information on the first clutch) as late-breeding pairs (i.e., pairs without local successful breeding experience, comprise pairs with failed/abandoned first broods). Only those pairs in the second/late breeding attempts that resulted in egg laying were included.
The phenology variables were encoded as the number of days that elapsed since the first of April (with day 1 = 1 April). The mean date that Japanese Tits laid their first egg was 24.14 ± 0.38 (n = 576 nests) in the first broods and 63.85 ± 0.95 (n = 60 nests) in the second broods. The mean dates that Eurasian Nuthatches, Daurian Redstarts, and Yellow-rumped Flycatchers laid their first egg were 22.05 ± 0.69 (n = 74 nests), 37.61 ± 2.75 (n = 49 nests), and 53.45 ± 0.54 (n = 240 nests), respectively. The clutch sizes of Japanese Tits’ first and second broods were 11.20 ± 0.07 (range: 6–16, n = 530 nests) and 8.05 ± 0.19 (range: 6–12, n = 60 nests), respectively. The clutch sizes of Eurasian Nuthatches, Daurian Redstarts, and Yellow-ridged Flycatchers were 6.90 ± 0.16 (range: 6–8, n = 65 nests), 6.50 ± 0.14 (range: 5–10, n = 46 nests) and 5.73 ± 0.06 (range: 4–8, n = 230 nests), respectively. The reproductive success rates of the first breeding Japanese Tits, Eurasian Nuthatches, Daurian Redstarts, and Yellow-ridged Flycatchers were approximately 81.25% (n = 576 nests), 87.84% (n = 74 nests), 87.86% (n = 49 nests), 79.58% (n = 240 nests), respectively.
From 2020 to 2021, a total of 192 pairs initiated first breeding attempts. To analyze whether Japanese Tit use social information from the previous year when choosing the nest site for their first breeding attempt, we randomly selected 192 empty nest boxes as control nests and counted whether first breeding and control nests had been occupied in the previous year.
From 2014 to 2021, a total of 63 pairs initiated second breeding attempts (i.e., were recaptured from the first breeding attempt) with the same mate (Table 1). Among the 63 second breeding pairs, three pairs had been recaptured in previous years. We excluded those three pairs to avoid duplication in subsequent analysis. In addition, 161 late-breeding pairs were monitored in the 8 years of the study (2014–2021; Table 1).
Year | Later breeder | Recaptured second breeder |
2014 | 0 | 11 |
2015 | 18 | 5 |
2016 | 15 | 3 |
2017 | 21 | 4 |
2018 | 31 | 7 |
2019 | 13 | 6 |
2020 | 37 | 19 |
2021 | 26 | 5 |
We mainly focused on available social information (i.e., the numbers of conspecifics and heterospecifics) around the nest sites that Japanese Tits selected for their second breeding attempt. We measured the Euclidean distance between the GPS points of nest sites during 2014–2021. The date of nest site selection (i.e., initiation of nest-building) cannot be clearly inferred from the data for each individual, but the date of onset of egg laying can be determined. Thus, we labeled the second breeding nests and late-breeding nests of Japanese Tits as the focal nest and then assessed the social information within a 200 m radius around this focal nest at the time of second breeding Japanese Tits’ own first egg-laying date. We chose 200 m for two reasons. First, the distance between the first and second nest sites of the 60 pairs of Japanese Tits recaptured within the same breeding season was <200 m (range: 20.1–182.9 m; mean ± standard error [SE] = 78.50 ± 6.77 m). Second, tits maintained a territory in our study area with a radius of approximately 200 m (Jin et al., 2021). We counted: 1) the number of breeding conspecific nests; 2) the number of breeding heterospecific nests; 3) the number of successful first nests of conspecifics (successfully fledged at least one young); 4) the number of successful heterospecific nests; 5) the number of failed first nests of conspecifics (no chicks have successfully fledged); and 6) the number of failed heterospecific nests.
In addition, for each pair of second breeding and late-breeding Japanese Tits, we randomly selected one unoccupied nest box (unoccupied by any birds) in the same forest patch within the corresponding year as the control nest box. Approximately 90% of these nest boxes were occupied by birds over the past seven years but none were occupied in the current year. We also assessed the social information within a 200 m radius around the control nest boxes. To be able to reliably compare social information available at the focal nest and at the control nest, this information needed to be assessed at the same time. Therefore, we monitored the same social information available 200 m around the control nest, on the first egg laying date of the focal nest. Data on the available social information around the control nest boxes were collected using the methods described above.
We used generalised linear mixed models (GLMM) with a logit link and binomial error distribution to test whether Japanese Tits in first breeding nest site selection would utilize social information from the previous year (“first-breeding nests” = 0, “control nests” = 1), with the explanatory variable being whether the same nest box (first breeding nest/control nest) was occupied in the previous year (“unoccupied” = 0, “occupied” = 1). Since some nest boxes were repeatedly occupied between years, “year” “ID of the nest box” and “ID of the forest patch” were added as random variables to avoid pseudo replication.
We used conditional logistic regression to compare social information around the second breeding nests with paired selected control (i.e., unoccupied) nests (“control nest” = 0, “occupied nest” = 1), where the identity of the Japanese Tit was the grouping variable (Koletsi and Pandis, 2017). We chose this approach because it is more appropriate and powerful than standard logistic regression for analyzing paired data such as nesting boxes vs. unoccupied boxes within a forest plot (Keating and Cherry, 2004; Bonaparte et al., 2020). The explanatory variables used in the models were: 1) the number of breeding conspecific nests; 2) the number of breeding heterospecific nests; 3) the number of successful first nests of conspecifics (we have deleted the nest made by the same pair); 4) the number of successful heterospecific nests; 5) the number of failed first nests of conspecifics; and 6) the number of failed heterospecific nests.
We also used conditional logistic regression to compare social information around the late-breeding nests with paired selected control (i.e., unoccupied) nests (“control nest” = 0, “late-breeding nest” = 1), where the identity of the Japanese Tit was the grouping variable. The explanatory variables were the six variables mentioned above.
To test whether successful breeding experience affected social information used, we used a GLMM with a logit link and binomial error distribution to compare social information around second breeding pairs and late-breeding pairs. The response variable was whether the artificial nest boxes were occupied by second breeding pairs or late-breeding pairs (“late-brooding pairs” = 0, “second breeding pairs” = 1). The explanatory variables were the six variables mentioned above. For the variable “successful first nests of conspecifics”, we have removed the nest made by the same pair. Since the data were collected in some forest patches over a span of several years, from 2014 to 2021, “year” and “ID of the forest patch” were added as random variables to avoid pseudo replication.
Explanatory variables used to build the models were checked for multicollinearity using Pearson's correlation matrix and variance inflation factors (VIFs). All explanatory variables displayed Pearson's correlation coefficient <0.65 (Table 2) and variance inflated factor (VIF) < 2) (O'Brien, 2007; Dyson et al., 2019), thus all fixed effects were retained in the final model. Model performance was evaluated using the area under the curve (AUC) of the receiver operating characteristic (ROC), a threshold-independent measure of the ability to distinguish existing points from random points (Peterson, 2006). Models with AUC values between 0.7 and 0.9 are considered good predictors (Pearce and Ferrier, 2000). The significance level was set at α = 0.05. All analyses were undertaken using the statistical software R 4.3.2 (R Core Team, 2023). We calculated AUC using the ‘ROCR’ package (Sing et al., 2005) and all P-values using Wald chi-square tests with the ANOVA function in the “car” package (Fox and Weisberg, 2019). Conditional logistic regression models were implemented using the clogit function found within the “survival” package (Therneau, 2015). The GLMMs were implemented using the package "lme4" (Bates et al., 2015).
Data of the second breeding nest of Japanese Tits (n=60) | ||||||
Breeding conspecific nests | Successful first nests of conspecifics | Failed first nests of conspecifics | Breeding heterospecific nests | Successful heterospecifics nests | Failed heterospecifics nests | |
Breeding conspecific nests | 1 | |||||
Successful first nests of conspecifics | 0.11 | 1 | ||||
Failed first nests of conspecifics | 0.54** | 0.22 | 1 | |||
Breeding heterospecific nests | 0.09 | 0.39** | 0.10 | 1 | ||
Successful heterospecifics nests | 0.02 | 0.47** | 0.15 | 0.40** | 1 | |
Failed heterospecifics nests | −0.17 | 0.01 | −0.10* | 0.31* | 0.03 | 1 |
Data of the 60 unoccupied nest | ||||||
Breeding conspecific nests | Successful first nests of conspecifics | Failed first nests of conspecifics | Breeding heterospecific nests | Successful heterospecifics nests | Failed heterospecifics nests | |
Breeding conspecific nests | 1 | |||||
Successful first nests of conspecifics | 0.06 | 1 | ||||
Failed first nests of conspecifics | 0.38** | 0.29* | 1 | |||
Breeding heterospecific nests | 0.16 | 0.14 | 0.16 | 1 | ||
Successful heterospecifics nests | −0.10 | −0.01 | 0.02 | 0.08 | 1 | |
Failed heterospecifics nests | −0.09 | −0.10 | −0.004 | −0.04 | 0.03 | 1 |
Data of the late-breeding nest of Japanese Tits (n=161) | ||||||
Breeding conspecific nests | Successful first nests of conspecifics | Failed first nests of conspecifics | Breeding heterospecific nests | Successful heterospecifics nests | Failed heterospecifics nests | |
Breeding conspecific nests | 1 | |||||
Successful first nests of conspecifics | −0.15 | 1 | ||||
Failed first nests of conspecifics | 0.05 | 0.00 | 1 | |||
Breeding heterospecific nests | 0.39** | 0.07 | −0.10 | 1 | ||
Successful heterospecifics nests | −0.13 | 0.22** | −0.06 | 0.05 | 1 | |
Failed heterospecifics nests | −0.01 | 0.01 | −0.02 | 0.19* | 0.10 | |
Data of the 161 unoccupied nest | ||||||
Breeding conspecific nests | Successful first nests of conspecifics | Failed first nests of conspecifics | Breeding heterospecific nests | Successful heterospecifics nests | Failed heterospecifics nests | |
Breeding conspecific nests | 1 | |||||
Successful first nests of conspecifics | −0.13 | 1 | ||||
Failed first nests of conspecifics | 0.25** | 0.22** | 1 | |||
Breeding heterospecific nests | 0.10 | 0.31** | 0.19* | 1 | ||
Successful heterospecifics nests | −0.08 | 0.11 | 0.28** | −0.02 | 1 | |
Failed heterospecifics nests | −0.12 | −0.03 | 0.06 | −0.09 | 0.13 | 1 |
Data of the second breeding nest of Japanese Tits (n=60) and unoccupied nest (n=60) | ||||||
Breeding conspecific nests | Successful first nests of conspecifics | Failed first nests of conspecifics | Breeding heterospecific nests | Successful heterospecifics nests | Failed heterospecifics nests | |
Breeding conspecific nests | 1 | |||||
Successful first nests of conspecifics | 0.12 | 1 | ||||
Failed first nests of conspecifics | 0.44** | 0.23* | 1 | |||
Breeding heterospecific nests | 0.13 | 0.29** | 0.12 | 1 | ||
Successful heterospecifics nests | 0.01 | 0.36** | 0.09 | 0.30** | 1 | |
Failed heterospecifics nests | −0.13 | −0.02 | −0.05 | 0.16 | 0.00 | 1 |
Data of the late-breeding nest of Japanese Tits (n=161) and unoccupied nest (n=161) | ||||||
Breeding Conspecific nests | 1 | |||||
Successful first nests of conspecifics | −0.13* | 1 | ||||
Failed first nests of conspecifics | 0.13* | 0.11* | 1 | |||
Breeding heterospecific nests | 0.30** | 0.18** | 0.04 | 1 | ||
Successful heterospecifics nests | −0.10 | 0.20** | 0.07 | 0.05 | 1 | |
Failed heterospecifics nests | −0.03 | 0.01 | 0.02 | 0.10 | 0.12* | 1 |
Data of the late-breeding (n=161) and second breeding nest (n=60) of Japanese Tits | ||||||
Breeding conspecific nests | 1 | |||||
Successful first nests of conspecifics | −0.10 | 1 | ||||
Failed first nests of conspecifics | 0.12 | 0.05 | 1 | |||
Breeding heterospecific nests | 0.29** | 0.21** | −0.04 | 1 | ||
Successful heterospecifics nests | −0.10 | 0.30** | −0.08 | 0.18** | 1 | |
Failed heterospecifics nests | −0.03 | 0.00 | −0.03 | 0.21** | 0.07 | 1 |
There was no significant difference between the probability that the Japanese Tit's first breeding nest was occupied in the previous year than control nest box (χ2 = 1.32, df = 1, p = 0.251).
Compared to the control nest boxes, the nest sites of Japanese Tits during the second breeding attempt (n = 60) had significantly more breeding conspecific nests (CLR: odds ratio (OR) = 13.76, 95% credible interval (CI) = [2.05, 92.55], χ2 = 4.66, df = 1, p < 0.03; Fig. 2A), more successful first nests of conspecifics (OR = 1.84, 95% CI = [1.12, 3.04], χ2 = 11.72, df = 1, p = 0.001; Fig. 2B), and fewer failed first nests of conspecifics (OR = 0.45, 95% CI = [0.18, 1.13], χ2 = 4.41, df = 1, p = 0.04; Fig. 2C) within a 200 m radius (Table 2). However, there was no difference in the breeding heterospecific nests (OR = 1.32, 95% CI = [0.77, 2.26], χ2 = 1.93, df = 1, p = 0.17; Fig. 2D), successful nests of heterospecifics (OR = 2.60, 95% CI = [0.78, 8.67], χ2 = 2.99, df = 1, p = 0.08; Fig. 2E), and failed nests of heterospecifics (OR = 1.24, 95% CI = [0.36, 4.29], χ2 = 0.11, df = 1, p = 0.74; Fig. 2F) between the second breeding nests and the control nest boxes. The model performance was excellent, as revealed by the AUC value of 0.85.
Compared to the control nest boxes, the nest sites of late-breeding Japanese Tits (n = 161) had significantly more breeding conspecific nests (CLR: OR = 1.48, 95% CI = [1.07, 2.04], χ2 = 3.78, df = 1, p = 0.05; Fig. 3A), more successful first nests of conspecifics (OR = 1.32, 95% CI = [1.02, 1.72], χ2 = 5.61, df = 1, p = 0.02; Fig. 3B), and more successful heterospecific nests (OR = 2.39, 95% CI = [1.40, 4.10], χ2 = 14.13, df = 1, p < 0.001; Fig. 3E) within a 200 m radius (Table 2). However, there was no difference in the breeding heterospecific nests (OR = 1.25, 95% CI = [0.89, 1.77], χ2 = 2.34, df = 1, p = 0.13; Fig. 3D), first breeding failed nests of conspecifics (OR = 1.14, 95% CI = [0.88, 1.47], χ2 = 2.16, df = 1, p = 0.14; Fig. 3C), and failed nests of heterospecific (OR = 2.07, 95% CI = [0.83, 5.18], χ2 = 2.97, df = 1, p = 0.08; Fig. 3F) between the late-breeding nests and the control nest boxes. The model performance was relatively good, as revealed by the AUC value of 0.72.
Second breeding pairs with successful breeding experience were surrounded by more successful first nests of conspecifics than were late-breeding pairs without successful breeding experience (GLMM: OR = 1.42, 95% CI = [1.12, 1.81], χ2 = 8.27, df = 1, p = 0.004; Fig. 4B). However, the number of breeding conspecific nests, the number of breeding heterospecific nests, the number of failed first nests of conspecifics, the number of successful heterospecific nests, and the number of first breeding failed heterospecific nests did not differ between the second breeding pairs and late-breeding pairs (p ≥ 0.10 for all, Fig. 4). The model performed well with an AUC value of 0.77.
In our study, Japanese Tits in their second breeding attempt seemed to be attracted to areas with more already settled breeding conspecifics. Our results were in line with studies of other bird species, such as the Golden-cheeked Warbler (Setophaga chrysoparia) and American Redstart (Setophaga ruticilla), which also showed a preference for using conspecific information in choosing nest sites (Fletcher, 2007; Farrell et al., 2012). This phenomenon is known as conspecific attraction (Doligez et al., 2002; Buxton and Sperry, 2020). The presence of conspecifics serves as an indirect signal of good habitat quality (Szymkowiak et al., 2016). In addition, settling around more conspecifics may reduce predation risk through dilution (Danchin and Wagner, 1997; Brown, 2016) and enhanced detection of nest threats (Roberts, 1996; Campobello and Sealy, 2011; Kelly et al., 2018). In our study, Japanese Tits are present as residents at breeding grounds year-round, and they have sufficient time to gain knowledge of the location of breeding conspecifics. Thus, we speculated that Japanese Tits might also follow a conspecific attraction strategy for selecting their second breeding nest sites. With so many available nest boxes (about 60% of nest boxes were unoccupied by birds), the emergence of this preference suggests that nest site selection was more than simply reselecting the dominant location. Therefore, the choice of second breeding individuals closer to the conspecifics is supposed to be a function of social information.
Japanese Tits in our study area seemed to rely on the reproductive success of conspecifics to select nest sites during their second breeding attempt. Previous studies using controlled trials and radio frequency identification (RFID) technology have shown that some species visit the nest boxes of other conspecifics throughout the breeding season to obtain information for future breeding decisions (Doligez et al., 2002, 2004; Firth and Sheldon, 2015; Schuett et al., 2017; Firth et al., 2018). Reproductively successful nests may be a particularly useful indicator of habitat quality because they represent the net effect of many different ecological and social factors that may each influence site quality (Danchin and Wagner, 1997). We have observed that Japanese Tits had prospected the nest boxes of other conspecifics using radio frequency identification (RFID) devices (Li et al., 2023). Thus, Japanese Tits tend to select nest sites in areas where there are many reproductively successful conspecific nests. In addition, our results found that Japanese Tits tended to select nest sites in areas where there were fewer failed conspecific nests in the second breeding attempt. In our study area, 71/105 monitored nests failed in the last 5 years (2017–2021), mainly due to predation. Predation is the major cause of total nest failure in passerine birds (Nilsson, 1984), and the selection of nest sites that reduce predation risk is thought to be the main driving factor for nest site selection in many birds (Martin, 1993).
In this study, we did not observe any heterospecific social information that could affect the nest site selection of recaptured second breeding Japanese Tits. This result may be explained by the fact that there were more conspecifics than heterospecifics in our study area. The occupancy rate of nest boxes by the other three species (approximately 13.4%) was much lower than that of Japanese Tits (approximately 26.3%). Jaakkonen et al. (2015) found that Collared Flycatchers (Ficedula albicollis) tended to rely on social cues provided by conspecifics when conspecific tutors were numerous in relation to heterospecific tutors. In addition, species are more likely to use heterospecific information only when it is difficult to obtain accurate information on local habitat quality from conspecifics (Seppänen et al., 2007). Therefore, our results support the idea that conspecific information is used by birds more available than heterospecific information.
Late-breeding individuals still tended to breed closer to already settled breeding conspecifics and were attracted to areas with a higher number of successful first nests of conspecifics. Unlike second breeders, the number of successfully heterospecific nests also influenced nest site selection for late-breeders but not the number of failed heterospecific nests. These later breeders may consist of individuals who moved into the study area, those who breed later than others, and those who breed in replacement nests (the nest produced after the deserted or predated of the former nest). Even though we could not exclude the possibility that the late breeders had engaged in breeding activities in the study area before, it was clear that they lacked local breeding success experience and/or had limited breeding time. The limited time windows for accessing information may prevent late breeders from fully observing the breeding activity and eventual fate of other individuals. The presence of conspecific and heterospecifics may represent a higher availability of food and fewer predators in this area (Forsman et al., 2007; Jaakkonen et al., 2015). Thus, late-breeders, with their lack of personal information, may rely on more source of information than the second breeding tits. Based on these findings, we suggest that Japanese Tits are flexible in their ability to use social information for nest site selection.
We found that Japanese Tits were not influenced by social information from the previous year when choosing a nest site for the first breeding attempt. Several studies have shown that some birds can use social cues from the previous year to influence breeding site selection the following year (Betts et al., 2008; Pärt et al., 2011). However, these conclusions were premised on the fact that these individuals breeding in this area have a high degree of study area (site) fidelity, and therefore social cues from the previous year can be utilised. Mortality or migration of Japanese Tits was high in our study area, with only 6.87% of ringed individuals recaptured the following year over a five year period. Therefore, tits didn't have social information from the previous year to assess nest site quality. During late April to early May when Japanese Tits were at the peak of their nest site selection for the first breeding attempt, they can only select nest sites using existing vegetation structure cues (Li et al., 2023).
Furthermore, we found that successful breeding experiences may influence how individuals use social information. Compared with that around late-breeding pairs, the number of successful conspecific nests around the second breeding pairs was higher. Previous studies have shown that breeding site experience affects nest site selection in territorial species (Serrano et al., 2001). For example, Lesser Kestrels (Falco naumanni) with successful breeding experience were more likely to breed near their original nest site than individuals without breeding experience, which could be related to benefits derived from increased local familiarity (Serrano et al., 2001). In this study, the second breeding tit pairs were more likely to have gained knowledge of local ecological conditions and the fate of neighbours during their first breeding attempt. Those tit pairs with successful breeding experience tend to choose a nest site in an area with more successful conspecific nests, which may represent a better habitat quality (Citta and Lindberg, 2007; Redmond et al., 2009). And it is possible that good quality habitats have aggregated local breeders, without the need of social information, or that social information favoured aggregation early on in the season and that prolonged to second breeding attempts, while late-breeders (Possibly less competitive, at least not so local) may not secure these good habitats.
Our results suggest that Japanese Tits can use social information from conspecifics and heterospecifics when choosing a nest site. All species that interact within a particular area are potential sources of information. Meanwhile, social cues may change over time, and their accessibility may also be time-bound. As resident birds, Japanese Tits may provide information about a breeding location earlier in the breeding season (on their first attempt) as well as scrounge information later in the breeding season (on their second attempt). Among acquired social information, Japanese Tits can rely on the most dependable information to select nest sites. This further illustrates that social information use is a subtle, complex and dynamic phenomenon. We suggest that further studies be conducted on diverse taxa to obtain a better understanding of the use of social information, especially in multiple-brooding species.
The present study complies with the current laws of China. Fieldwork was carried out with permission from the Zuojia Nature Reserve, Jilin, China. Experimental procedures were permitted by the National Animal Research Authority of Northeast Normal University (Approval Number: NENU-20080416) and the Forestry Bureau of Jilin Province of China (Approval Number: [2006]178).
Data areavailable from the Dryad Digital Repository: https://doi.org/10.5061/dryad.rfj6q57gh.
Xudong Li: Writing – original draft, Software, Methodology, Investigation, Formal analysis, Data curation. Jiangping Yu: Writing – review & editing, Funding acquisition, Formal analysis, Conceptualization. Dake Yin: Investigation. Longru Jin: Writing – review & editing, Data curation, Conceptualization. Keqin Zhang: Writing – review & editing, Investigation, Funding acquisition, Conceptualization. Li Shen: Writing – review & editing, Investigation, Data curation, Conceptualization. Zheng Han: Writing – review & editing, Methodology, Conceptualization. Haitao Wang: Writing – review & editing, Funding acquisition, Formal analysis, Conceptualization, Li Xudong, Writing – original draft, Software, Methodology, Investigation, Formal analysis, Data curation.
The authors declare that they have no known competing financial interests or personal relationships that could have appeared to influence the work reported in this paper.
We wish to thank all people who collaborated on the data collection: Ye Gong, Qianxi Fan, Hongwei Xu, Jingnan Liang, Jing Yue, Can Sun, Hailin Lu, Na Zhao, Liufang Wang, Jian Fang, Junlong Yin, Yimo Yang, Anli Zhang, and many others. Fieldwork was performed with the permission of the Nature Reserve of Zuojia.
Firth, J.A., Sheldon, B.C., 2015. Experimental manipulation of avian social structure reveals segregation is carried over across contexts. Proc. R. Soc. B 282, 165–183.
|
Kendal, R.L., Coolen, I., van Bergen, Y., Laland, K.N., 2005. Trade‐offs in the adaptive use of social and asocial learning. Adv. Stud. Behav. 35, 333–379.
|
Melles, S., Glenn, S.M., Martin, K., 2003. Urban bird diversity and landscape complexity: species-environment associations along a multiscale habitat gradient. Conserv. Ecol. 7, 5.
|
Podofillini, S., Cecere, J.G., Griggio, M., Curcio, A., De Capua, E.L., Fulco, E., et al., 2018. Home, dirty home: effect of old nest material on nest-site selection and breeding performance in a cavity-nesting raptor. Curr. Zool. 64, 693–702.
|
R Core Team, 2023. R: a Language and Environment for Statistical Computing.
|
Safran, R.J., 2006. Nest-site selection in the barn swallow, Hirundo rustica: what predicts seasonal reproductive success? Can. J. Physiol. Pharmacol. 84, 1533–1539.
|
Year | Later breeder | Recaptured second breeder |
2014 | 0 | 11 |
2015 | 18 | 5 |
2016 | 15 | 3 |
2017 | 21 | 4 |
2018 | 31 | 7 |
2019 | 13 | 6 |
2020 | 37 | 19 |
2021 | 26 | 5 |
Data of the second breeding nest of Japanese Tits (n=60) | ||||||
Breeding conspecific nests | Successful first nests of conspecifics | Failed first nests of conspecifics | Breeding heterospecific nests | Successful heterospecifics nests | Failed heterospecifics nests | |
Breeding conspecific nests | 1 | |||||
Successful first nests of conspecifics | 0.11 | 1 | ||||
Failed first nests of conspecifics | 0.54** | 0.22 | 1 | |||
Breeding heterospecific nests | 0.09 | 0.39** | 0.10 | 1 | ||
Successful heterospecifics nests | 0.02 | 0.47** | 0.15 | 0.40** | 1 | |
Failed heterospecifics nests | −0.17 | 0.01 | −0.10* | 0.31* | 0.03 | 1 |
Data of the 60 unoccupied nest | ||||||
Breeding conspecific nests | Successful first nests of conspecifics | Failed first nests of conspecifics | Breeding heterospecific nests | Successful heterospecifics nests | Failed heterospecifics nests | |
Breeding conspecific nests | 1 | |||||
Successful first nests of conspecifics | 0.06 | 1 | ||||
Failed first nests of conspecifics | 0.38** | 0.29* | 1 | |||
Breeding heterospecific nests | 0.16 | 0.14 | 0.16 | 1 | ||
Successful heterospecifics nests | −0.10 | −0.01 | 0.02 | 0.08 | 1 | |
Failed heterospecifics nests | −0.09 | −0.10 | −0.004 | −0.04 | 0.03 | 1 |
Data of the late-breeding nest of Japanese Tits (n=161) | ||||||
Breeding conspecific nests | Successful first nests of conspecifics | Failed first nests of conspecifics | Breeding heterospecific nests | Successful heterospecifics nests | Failed heterospecifics nests | |
Breeding conspecific nests | 1 | |||||
Successful first nests of conspecifics | −0.15 | 1 | ||||
Failed first nests of conspecifics | 0.05 | 0.00 | 1 | |||
Breeding heterospecific nests | 0.39** | 0.07 | −0.10 | 1 | ||
Successful heterospecifics nests | −0.13 | 0.22** | −0.06 | 0.05 | 1 | |
Failed heterospecifics nests | −0.01 | 0.01 | −0.02 | 0.19* | 0.10 | |
Data of the 161 unoccupied nest | ||||||
Breeding conspecific nests | Successful first nests of conspecifics | Failed first nests of conspecifics | Breeding heterospecific nests | Successful heterospecifics nests | Failed heterospecifics nests | |
Breeding conspecific nests | 1 | |||||
Successful first nests of conspecifics | −0.13 | 1 | ||||
Failed first nests of conspecifics | 0.25** | 0.22** | 1 | |||
Breeding heterospecific nests | 0.10 | 0.31** | 0.19* | 1 | ||
Successful heterospecifics nests | −0.08 | 0.11 | 0.28** | −0.02 | 1 | |
Failed heterospecifics nests | −0.12 | −0.03 | 0.06 | −0.09 | 0.13 | 1 |
Data of the second breeding nest of Japanese Tits (n=60) and unoccupied nest (n=60) | ||||||
Breeding conspecific nests | Successful first nests of conspecifics | Failed first nests of conspecifics | Breeding heterospecific nests | Successful heterospecifics nests | Failed heterospecifics nests | |
Breeding conspecific nests | 1 | |||||
Successful first nests of conspecifics | 0.12 | 1 | ||||
Failed first nests of conspecifics | 0.44** | 0.23* | 1 | |||
Breeding heterospecific nests | 0.13 | 0.29** | 0.12 | 1 | ||
Successful heterospecifics nests | 0.01 | 0.36** | 0.09 | 0.30** | 1 | |
Failed heterospecifics nests | −0.13 | −0.02 | −0.05 | 0.16 | 0.00 | 1 |
Data of the late-breeding nest of Japanese Tits (n=161) and unoccupied nest (n=161) | ||||||
Breeding Conspecific nests | 1 | |||||
Successful first nests of conspecifics | −0.13* | 1 | ||||
Failed first nests of conspecifics | 0.13* | 0.11* | 1 | |||
Breeding heterospecific nests | 0.30** | 0.18** | 0.04 | 1 | ||
Successful heterospecifics nests | −0.10 | 0.20** | 0.07 | 0.05 | 1 | |
Failed heterospecifics nests | −0.03 | 0.01 | 0.02 | 0.10 | 0.12* | 1 |
Data of the late-breeding (n=161) and second breeding nest (n=60) of Japanese Tits | ||||||
Breeding conspecific nests | 1 | |||||
Successful first nests of conspecifics | −0.10 | 1 | ||||
Failed first nests of conspecifics | 0.12 | 0.05 | 1 | |||
Breeding heterospecific nests | 0.29** | 0.21** | −0.04 | 1 | ||
Successful heterospecifics nests | −0.10 | 0.30** | −0.08 | 0.18** | 1 | |
Failed heterospecifics nests | −0.03 | 0.00 | −0.03 | 0.21** | 0.07 | 1 |