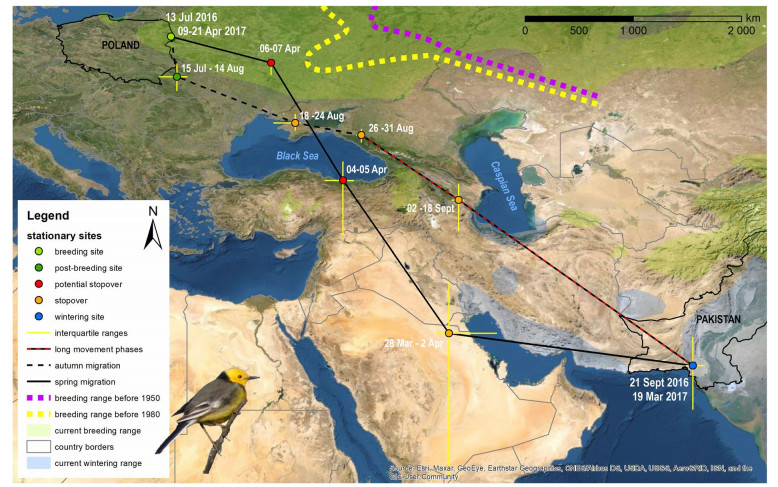
Citation: | Bertille Mohring, François Brischoux, Frédéric Angelier. 2021: Vineyards, but not cities, are associated with lower presence of a generalist bird, the Common Blackbird (Turdus merula), in Western France. Avian Research, 12(1): 3. DOI: 10.1186/s40657-020-00239-0 |
Land-use change is one of the main drivers of the global erosion of biodiversity. In that context, it is crucial to understand how landscape characteristics drive the presence of rare endangered species. Nevertheless, it is also important to study common species in multiple habitats, because they represent a large proportion of biodiversity and are essential to maintain ecological functions. Interestingly, some habitats, as farmlands with permanent crops (e.g. vineyards), have been overlooked in the literature.
In this study, we investigated the distribution of a widespread and common bird species, the Common Blackbird (Turdus merula), within and between the three main habitats of our study area (rural Western France). We specifically focused on (1) woodlands, (2) farmlands with a high vineyard coverage, and (3) moderately urbanized areas. Specifically, we aimed to assess the beneficial and detrimental effects of these habitats and their fine-scale composition on the presence of a common bird species, relying on a survey by point counts (nearly 100 locations). We studied the effects of habitats and gradients of fine-scale habitat composition on blackbird presence using logistic regression analyses.
Blackbirds were present in all studied habitats. However, their presence varied between habitats, being lower in vineyards than in woodlands and cities. In woodlands and cities, fine-scale analyses did not reveal any component driving the species' presence. However, we found that shrub and tree vegetation cover had a significant positive effect on blackbird presence in vineyards.
Our results are in agreement with the definition of a generalist species. Interestingly, species distribution varied between habitats. The high presence of blackbirds in urban areas suggests that medium-sized cities, despite their artificialization, do not constrain the settlement of this former forest specialist and that green spaces may allow blackbirds to thrive in medium-sized cities. On the contrary, we found an impoverished presence of blackbirds in vineyards and a positive effect of vegetation on their presence in these landscapes. This suggests that permanent crops, and more generally farmlands, may impose important constraints to common species. Future studies should examine how to enhance biodiversity through agricultural management policies, especially in vineyards.
Most long-distance migrants of the western Palaearctic spend winters in Africa (Briedis et al. 2020a), whereas very few bird species migrate eastward to spend the non-breeding period in South Asia (Newton 2008). This fact reflects a little attention to this flyaway in the literature (Lislevand and Hahn 2015; Stach et al. 2016; Brlík et al. 2018; Lisovski et al. 2021). Also the recoveries of ringed birds remained scarce and deficient for describing the Indo-European flyway (Lisovski et al. 2021). This flyway is however unique as it is utilized by many bird species that are currently extending their breeding ranges towards western Europe (del Hoyo et al. 2005). Migration routes often reflect historical expansions of bird populations (Wiltschko and Wiltschko 2003; Bairlein et al. 2012). This was found recently in passerines that winter in India sub-continent (Stach et al. 2016; Brlík et al. 2018). Studying migration of the species that utilize Indo-European flyway can therefore provide information about the course of the breeding population expansion, mechanisms underlying the migration routes evolution and knowledge about key stopover and wintering areas within that system.
The Citrine Wagtail (Motacilla citreola) is a passerine that gradually expanded its breeding range from Asia westward to areas in central and western Europe (Meissner and Skakuj 1997) and is an example of a species that had has recently lengthened its migration route (Newton 2008). In last 60 years, the breeding range of the subspecies werae has extended westward more than 1000 km, from the Moscow region to all central European countries (Dementiev and Gladkov 1954; Keller et al. 2020), where it currently breeds regularly (Ściborska 2004; Krajewski 2016). The Citrine Wagtail population from north Siberia winters from south of the Caspian Sea and southern Iran to India (Cramp 1998), while birds from central European Russia migrate eastward from the Caspian Sea through Turkmenistan, eastern Iran and Afghanistan to spend winter on the Indian sub-continent (Ali and Ripley 1973). However, information regarding Citrine Wagtail migration routes between breeding sites in central Europe and wintering sites is almost completely lacking (see: Hnatyna et al. 2020). The aim of this study was to use light-level loggers to reveal the Citrine Wagtail migratory pattern and to identify stopovers and wintering quarters.
The fieldwork was carried out in north Poland (52.98° N, 23.70° E) where within a habitat of around 50 ha (rewetted degraded peatland) 4 to 8 pairs of Citrine Wagtails breed regularly (Tumiel et al. 2013). In June 2016, we trapped 9 individuals (6 males and 3 females) that we ringed and equipped with light-level geolocators (0.6 g Intigeo-P50Z11-7, Migrate Technology, Cambridge, UK) by attachment to the bird's rump with a flexible 0.8 mm thick leg-loop harness (Rappole and Tipton 1991). In 2017, we recovered one geolocator from a previously tagged male and one more male with a tag was seen in the field with a plastic ring (not retaped), indicating that at least two different tagged individuals had returned (22% of tagged birds).
To analyse the light-level data we used a threshold approach in R package 'GeoLight' (Lisovski and Hahn 2012; R Core Team 2018). To determine sun elevation angle (at a light threshold value = 2) we used 'getElevation' function (based one light recordings from the breading site after tag deployment) and Hill–Ekstrom calibration (Lisovski and Hahn 2012; Lisovski et al. 2020) of the longest stationary site (wintering site). This resulted in obtaining − 3.2° and − 4° sun elevation angle, respectively. We decide to apply a mean value of those (SEA = − 3.6) to calculate the coordinates for the non-breeding period. Stationary periods were classified using the 'changeLight' function (change probability q = 0.8) and a minimum stationary period of 3 days (Lisovski et al. 2020). In order to avoid stationary periods separation caused by single large errors in the twilight, we used 'mergeSites' function to finally estimate stopover (distance threshold = 150 km) and wintering sites (distance threshold = 300 km). However, due to a lack of long stationary periods on the spring migration (only one was longer than 3 days), in two instances we also calculated two potential stopovers shorter than 3 days assigning them as potential stopovers (Fig.1). The presence of those stopovers were found by visual inspection of latitude and longitude change over time as well as a 'changeLight' function with minimum stationary period of 1 day. The arrival and departure timing and movement periods were determined by inspecting patterns of latitude and longitude over time (Lindström et al. 2016). Long movement phases were classified as part of a migration between stationary sites longer than 24 h with continuous longitudinal changes over time and no stopovers identified. Maps were created in GIS (ArcGIS 10.1, Redlands, CA, USA) based on stationary periods and errors (interquartile ranges) estimated in 'mergeSites' function.
We calculated a great circle distances between successive stationary sites (see Additional file 1: Table S1) and the migration distance as the sum of those distances between the breeding and wintering grounds (Table 1). The migration duration was calculated as the total number of days spent on migration (between departure and arrival dates), whereas the duration of movement was considered as the number of days spent moving, excluding stopover periods. Movement speed (km/day) was calculated as the distance between stopover positions divided by the number of movement days, whereas migration speed (km/day) was determined as the migration distance divided by the total number of days spent on migration, including stopover periods.
From | Departure date | Arrival date | Migration duration (days) | Number of stopovers | Duration of movement (days) | Stopover duration (days) | Distance (km) | Migration speed (km/day) | Movement speed (km/day) |
Autumn migration | |||||||||
Breeding site | 2016-07-13 | 2016-09-21 | 70 | 4 | 13.5 | 56.5 | 4800 | 69 | 356 |
Post-breeding site | 2016-08-14 | 2016-09-21 | 38.5 | 3 | 12 | 26.5 | 4450 | 116 | 371 |
Spring migration | |||||||||
Wintering grounds | 2017-03-19 | 2017-04-091 | 21.5 | 3 | 14 | 7.5 | 5640 | 262 | 403 |
2017-03-19 | 2017-04-212 | 33 | > 3 | 27.5 | 22 | 5640 | 171 | 205 | |
Distances are reportd to nearest 10 km. Dates in the end of spring migration is partly missing due to improper light measurements (see methods) thus the 1 arrival date is the date 2 days after the last stopover site position while the 2 is the first light logger position at the breeding sites when the logger starts working again. Arrival dates indicate the presumed period when the individual arrived to breeding grounds |
The studied individual left the breeding site on 13 July and in one and a half days moved south to west Ukraine where it spent 30 days. Given that this first stop (post breeding site, Fig.1) was fairly long and the estimated positions of that site were based on a high variation in latitude in that period (because of irregular pattern in light measurements), we interpret this stopover as a dispersal towards post-breeding moulting and fuelling sites. Our conclusion is based on Hnatyna et al. (2020) who found that in western Ukraine half of the captured Catrine Wagtail adults at that time of the year were in various stages of active moult and fat deposition. The authors estimate that the moult lasts around one month and thus starts after mid-July which fits into timing of the post-breeding site we distinguished (15 July–14 August). Moreover during the moult period birds often make night roosts in reed beds (Hnatyna et al. 2020) what may explain a different pattern in light measurements and so high variation in positions estimates (Lisovski et al. 2012, 2020). We therefore reported migration parameter estimations based on both departure dates from the breeding site and from the post-breeding site (Table 1). After 14 August, the studied bird started a fast migration, proceeding in a southeast direction and making 2 stopovers (in a total of 16 days, including movement: 15–31 August) along the north coast of the Black Sea (Fig.1). From there, in 48 h, the bird performed the first long movement covering a distance of 920 km (mean movement speed = 460 km/day, Table 2), reaching north Iran, where it made a prolonged stop of 16 days. On 18 September the studied Citrine Wagtail performed a second fast (mean movement speed = 640 km/day, Table 2) and long movement phase (2240 km in 84 h, Table 2) crossing the arid landscapes in Iran and Pakistan until arriving on 21 September, at the lower Indus River valley in Pakistan. This endurance flight indicates that the preceding stopover (that was the longest on migration) had a fuel deposition meaning to allow crossing the arid landscape. The autumn migration distance was 4800 km (duration 70 days) with a migration speed of 69 km/day when considering the post-breeding site as a first stopover. Excluding it, the migration distance covered 4450 km (duration 38.5 days) with an average migration speed of 116 km/day (Table 1). The studied individual wintered in south Pakistan close to or within the Indus river delta (duration 178.5 days). The spring migration started on 19 March following a more southern route back to the breeding site than it had taken in the autumn migration (Fig.1). The first 9 days of the journey were in progressive movement phases westward (1860 km) with a relatively slow movement speed of 207 km/day (Additional file 1: Table S1), which suggests that, at this part of the journey the Citrine Wagtail was foraging and flying in an intermittent migratory strategy. After that, the studied male made the only long stopover during the spring migration (5 days: 28 March–02 April), potentially in the Tigris-Euphrates delta (the latitude of this site is highly uncertain because of closeness to Equinox [Lisovski et al. 2012]). From that site, the Citrine Wagtail continued a fast journey, heading in a northwest direction, with a short stop in Turkey (a potential stopover 1.5 days, Fig.1) afterwards heading north (potentially crossing the Black Sea) where it made a short stop for at least one day (6–7 April). However the latitudes of those position may be uncertain while estimated based on four and two positions respectively. Afterwards (08–21 April), the tag recorded the light improperly (permanent full light range). The estimated positions after the light recording error (from 21 to 27 April) were around the breeding sites (after that time, the tag was no longer recording), indicating that the spring migration had finished within the period between 9 and 21 April (assuming at least 48 h to get to the breeding sites from the last spring stopover) and covered 5640 km (Table 1). According to this assumption, the spring migration duration was between 21.5 and 33 days, which gives a migration speed between 171 and 262 km/day. However, while the average spring migration speed until the last stopover before the tag record light improperly was around 270 km/day (Additional file 1: Table S1), the second value of the overall spring migration speed seems to be more reasonable because it is very unlikely that at the end of migration (650 km from the breeding grounds) a male would change the migration behaviour and move relatively slowly with an average speed of 57 km/day, especially given that the early arrival of males to the breeding grounds is highly beneficial (explained below). We therefore use spring migration speed 262 km/day in further discussions.
No | Departure | Arrival | Duration (h) | Distance (km) | Movement speed | |
km/day | m/s | |||||
1 | 2016-08-31 | 2016-09-02 | 48 | 920 | 460 | 5.32 |
2 | 2016-09-18 | 2016-09-21 | 84 | 2240 | 640 | 7.41 |
Distances are reportd to nearest 10 km |
The overall spring migration speed (262 km/day) of the studied individual was approximately twice as fast as the autumn migration speed (116 km/day) and was a consequence of a longer stopover duration in the autumn (26.5 days in autumn vs. 7.5 days in spring, in total). A faster spring migration is also observed in many other long-distance migrants, since early arrival at the breeding grounds allows the establishment of higher quality territories and increases the chances of finding a mate, thereby relating to a higher fitness (Moore et al. 2005; Nilsson et al. 2013). This pattern was also the case for the other individuals studied so far on the Indo-European flyway (Lislevand et al. 2015; Stach et al. 2016; Lisovski et al. 2021).
Within the overall migration of the studied Citrine Wagtail male, we distinguished two long movement phases covering a distance of 920 and 2240 km in autumn (30% of the whole journey length), with an average speed 574 km/day. We explain this rapid migration behaviour by the need to cross ecological barriers with limited refuelling possibilities in the Caucasian Region and the arid landscapes of the mountains and deserts in Iran (Kavir and Dasht-e Lut Desert) and Pakistan (Kharan Desert). The lack of clear long movement phases in the spring migration were presumably related to better feeding conditions in spring than in the autumn, since the precipitation in that region is lower in autumn and thus potential food resources for insectivorous bird species are very limited (Dolnik 1990; Lisovski et al. 2021).
The longest movement phase (84 h, Table 2) took place during the autumn migration when the Citrine Wagtail crossed the arid landscapes of Iran and Pakistan at a distance of 2240 km (640 km/day). This journey covered 3 days and 5 nights. Assuming that the ground flight speed of the Citrine Wagtail is similar to Pied Wagtail (Motacilla alba) (13.3 m/s, Pennycuick et al. 2013), the studied individual had to fly for about 47 h (56% of this movement phase) at maximum speed to cover that distance (no wind constraint/support consideration). In that long movement phase, the maximum day light was 37 h and 53 min (time with any light intensity recorded by the tag at this part of the journey). This suggests that the studied individual most likely also migrated at night to cover that distance. It is surprising while in general wagtails are considered to be a diurnal migrants (Alerstam and Ulfstrand 1975; Biebach et al. 2000; Schmaljohann et al. 2007a), and this also confirms behavioural observations of the Citrine Wagtail: they migrate in the daytime with yellow wagtails flock and use collective night roosting sites in reed beds (Zavyalov et al. 2008; Hnatyna et al. 2020). A prolonged nocturnal migration of an assumed diurnal migrant was also recently found for the Tawny Pipit (Anthus campestris), as more than 2/3 of all its migratory movements were carried out at night (Briedis et al. 2020b). Given that nocturnal migration is beneficial for birds due to a reduction in dehydration risks caused by high temperatures when crossing the deserts (Biebach 1990), and that flying non-stop in the daytime excludes the possibility of recovering energy and water reserves at small oases along the way (Schwilch et al. 2002), a nocturnal migration of assumed diurnal migrants may be a more common phenomenon than is currently recognised (Briedis et al. 2020b). We suggest that the mixed day-night migratory strategy (Schmaljohann et al. 2007b; Newton 2008) could represent a flexible migration strategy where prolonged flights were brought about by the need to cross ecological barriers (Schmaljohann et al. 2007b; Adamík et al. 2016)—in our example, a desert in Iran and Pakistan.
During the autumn and spring migration, the studied Citrine Wagtail flew south from the Caspian Sea. This migration course was unexpected while the migration routes of a Citrine Wagtail described previously (Cramp 1998) runs through a well-known passerine passage in the Central Asian region east of the Caspian Sea (Dolnik 1990; Chernetsov et al. 2007a). This migration route also retraced Citrine Wagtail breeding range expansion (Meissner and Skakuj 1997; Ściborska 2004) observed in the last decades (see the breeding range changes on Fig.1). A new migration route found here may therefore reflect an alternative expansion direction of Citrine Wagtail to Europe. If so, this suggests that breeding Citrine Wagtails found in central and western Europe may originate from a different historical subpopulation.
The possible presence of alternative migrating routes that suggests different population expansion directions and a mixed day-night migratory strategy makes the migration of the Citrine Wagtail interesting to study in future, especially with recent availability of multi-sensor tracking devices (Bäckman et al. 2017; Liechti et al. 2018).
The online version contains supplementary material available at https://doi.org/10.1186/s40657-021-00305-1.
Additional file 1: Table S1. The stationary sites along the migration route of the Citrine Wagtail male between the breeding site in Polamd to nonbreeding stationary sites on the Indian sub-continent.
We thank to Raymond H. G. Klaassen for advice on data analysis and general comments and Dorota Łukasik for support in obtaining a permit for this study from General Directorate for Environmental Protection. We thank the journal editors Lei Cao, a refere Martins Briedis and an anonymous refere for helpful comments and suggestions on previous versions of the manuscript.
MK and TT conceived and designed the study. MK, PB, GG, TT, PŚ and MW carried out fieldwork. MK analysed and interpreted the data. The manuscript was drafted by MK with input from TT, PB and PŚ. All authors read and approved the final manuscript.
The datasets used in the present study are available from the corresponding author on reasonable request.
Our research adheres to local guidelines and appropriate ethical approval and licences were obtained.
Not applicable.
The authors declare that they have no competing interests.
Abs M, Bergen F. A long term survey of the avifauna in an urban park. In: Marzluff JM, Shulenberger E, Endlicher W, Alberti M, Bradley G, Ryan C, et al., editors. Urban ecology: an international perspective on the interaction between humans and nature. Boston: Springer; 2008. p. 373–6.
|
Assandri G, Bogliani G, Pedrini P, Brambilla M. Insectivorous birds as 'non-traditional' flagship species in vineyards: applying a neglected conservation paradigm to agricultural systems. Ecol Indic. 2017a;80: 275–85.
|
Assandri G, Giacomazzo M, Brambilla M, Griggio M, Pedrini P. Nest density, nest-site selection, and breeding success of birds in vineyards: management implications for conservation in a highly intensive farming system. Biol Conserv. 2017b;205: 23–33.
|
Baillie J, Hilton-Taylor C, Stuart SN. IUCN red list of threatened species: a global species assessment. Gland: IUCN–The World Conservation Union; 2004.
|
Buckley NJ. The new atlas of breeding birds in Britain and Ireland: 1988–1991. In: Gibbons DW, Reid JB, Chapman RA, editors. The Auk. London: T & AD Poyser; 1995. p. 812–3.
|
Burnham KP, Anderson DR. Model selection and multi-model inference: a practical information-theoretic approach. 2nd ed. New York: Springer; 2002.
|
Clavel J, Julliard R, Devictor V. Worldwide decline of specialist species: toward a global functional homogenization? Front Ecol Environ. 2011;9: 222–8.
|
Dabelsteen T. Interactive playback: a finely tuned response. In: McGregor PK, editor. Playback and studies of animal communication. Boston: Springer; 1992. p. 97–109.
|
Fernández-Juricic E, Jimenez MD, Lucas E. Bird tolerance to human disturbance in urban parks of Madrid (Spain): management implications. In: Marzluff JM, Bowman R, Donnelly R, editors. Avian ecology and conservation in an urbanizing world. Boston: Springer; 2001. p. 259–73.
|
Guittet M, Sibe V, Gaudin J-C. Les vignobles: de nouveaux réservoirs de biodiversité. Faune sauvage. 2011: 9.
|
Hatchwell BJ, Chamberlain DE, Perrins CM. The demography of Blackbirds Turdus merula in rural habitats: is farmland a sub-optimal habitat? J Appl Ecol. 1996;33: 1114.
|
Lê S, Josse J, Husson F. FactoMineR: an R package for multivariate analysis. J Stat Softw. 2008;25: 1–18.
|
Lennon JJ, Beale CM, Reid CL, Kent M, Pakeman RJ. Are richness patterns of common and rare species equally well explained by environmental variables? Ecography. 2011;34: 529–39.
|
Luck GW, Smallbone LT. Species diversity and urbanisation: patterns, drivers and implications. In: Gaston KJ, editor. Urban Ecology. Cambridge: Cambridge University Press; 2010. p. 88–119.
|
Luniak M. Synurbization — adaptation of animal wildlife to urban development. In: Shaw WW, Harris LK, Vandruff L, editors. Proceedings 4th international urban wildlife symposium. Tucson, Arizona: University of Arizona; 2004. p. 50–5.
|
Mennechez G, Clergeau P. Effect of urbanisation on habitat generalists: starlings not so flexible? Acta Oecol. 2006;30: 182–91.
|
Pithon JA, Beaujouan V, Daniel H, Pain G, Vallet J. Are vineyards important habitats for birds at local or landscape scales? Basic Appl Ecol. 2016;17: 240–51.
|
R Core Team. R: A language and environment for statistical computing. Vienna, Austria: R Foundation for Statistical Computing; 2019.
|
Ralph CJ, Droege S, Sauer JR. Managing and monitoring birds using point counts: standards and applications. In: Ralph CJ, Droege S, Sauer JR, editors. Monitoring bird populations by point counts. Albany: U.S. Department of Agriculture, Forest Service, Pacific Southwest Research Station; 1995. p. 161–8.
|
Rotenberry JT, Wiens JA. A synthetic approach to principal component analysis of bird/habitat relationships. In: Capen DE, editor. The use of multivariate statistics in studies of wildlife habitat. Fort-Collins: Rocky Mountain Forest and Range Experiment Station; 1981. p. 197–208.
|
1. | Francisco Guil, Juan Manuel Pérez-García. Bird electrocution on power lines: Spatial gaps and identification of driving factors at global scales. Journal of Environmental Management, 2022, 301: 113890. DOI:10.1016/j.jenvman.2021.113890 |
2. | Binaya Adhikari, Shivish Bhandari, Kedar Baral, et al. Raptors at risk: Attributes of mortality within an anthropogenic landscape in the Mid-Hills region of Nepal. Global Ecology and Conservation, 2022, 38: e02258. DOI:10.1016/j.gecco.2022.e02258 |
From | Departure date | Arrival date | Migration duration (days) | Number of stopovers | Duration of movement (days) | Stopover duration (days) | Distance (km) | Migration speed (km/day) | Movement speed (km/day) |
Autumn migration | |||||||||
Breeding site | 2016-07-13 | 2016-09-21 | 70 | 4 | 13.5 | 56.5 | 4800 | 69 | 356 |
Post-breeding site | 2016-08-14 | 2016-09-21 | 38.5 | 3 | 12 | 26.5 | 4450 | 116 | 371 |
Spring migration | |||||||||
Wintering grounds | 2017-03-19 | 2017-04-091 | 21.5 | 3 | 14 | 7.5 | 5640 | 262 | 403 |
2017-03-19 | 2017-04-212 | 33 | > 3 | 27.5 | 22 | 5640 | 171 | 205 | |
Distances are reportd to nearest 10 km. Dates in the end of spring migration is partly missing due to improper light measurements (see methods) thus the 1 arrival date is the date 2 days after the last stopover site position while the 2 is the first light logger position at the breeding sites when the logger starts working again. Arrival dates indicate the presumed period when the individual arrived to breeding grounds |
No | Departure | Arrival | Duration (h) | Distance (km) | Movement speed | |
km/day | m/s | |||||
1 | 2016-08-31 | 2016-09-02 | 48 | 920 | 460 | 5.32 |
2 | 2016-09-18 | 2016-09-21 | 84 | 2240 | 640 | 7.41 |
Distances are reportd to nearest 10 km |